Chromium(VI) Oxidation of Cadaverine in Different Acidic Media: A Comparative Kinetic Study
Fawzy A.1, 2, *, Zaafarany I. A.1, Abdallah M.1, 3, Bawazeer T. M.1, Jassas R. S.1, Obaid R. J.1
1Chemistry Department, Faculty of Applied Sciences, Umm Al-Qura University, Makkah, Saudi Arabia
2Chemistry Department, Faculty of Science, Assiut University, Assiut, Egypt
3Chemistry Department, Faculty of Science, Benha University, Benha, Egypt
Email address

(Fawzy A.)
*Corresponding author
Citation
Fawzy A., Zaafarany I. A., Abdallah M., Bawazeer T. M., Jassas R. S., Obaid R. J. Chromium(VI) Oxidation of Cadaverine in Different Acidic Media: A Comparative Kinetic Study. Biochemistry and Molecular Biology. Vol. 1, No. 4, 2016, pp. 20-26.
Abstract
Kinetics of oxidation of cadaverine by chromium(VI) in aqueous perchloric and sulfuric acids media has been investigated spectrophotometrically at fixed ionic strength and temperature. In both acids the reactions showed a first order dependence on [Cr(VI)], less than unit order dependence with respect to cadaverine concentration. The effect of hydrogen ion concentration on the oxidation rates showed that the oxidation reactions in both acids were acid-catalyzed with fractional-second order dependences with respect to [H+]. The oxidation rates were not affected significantly by variation of ionic strength or dielectric constant of the reactions media. Addition of Mn(II) was found to decrease the oxidation rates. In both acids, the final oxidation products of cadaverine were identified as 5-aminopentanal and ammonia. Under comparable experimental conditions, the oxidation rate of cadaverine in sulfuric acid was found to be higher than that in perchloric acid. The plausible oxidation mechanism were proposed and the rate-law expression was derived. The activation parameters with respect to the second order rate constants were evaluated.
Keywords
Cadaverine, Chromium(VI), Oxidation, Kinetics, Mechanism
1. Introduction
Cadaverine (1,5-pentanediamine) is a foul-smelling toxic diamine compound produced by protein hydrolysis during putrefaction of animal tissue [1]. It is produced in small quantities by living beings. It is partially responsible for the distinctive odors of urine [2]. Elevated levels of cadaverine have been found in the urine of some patients with defects in lysine metabolism. It is also presents in the coastal seawater. Scanning the literature showed a lack of information as to the kinetic studies on the oxidation of cadaverine.
Chromium(III) and chromium(VI) oxidation states of chromium are characterized by different physical/chemical behavior and toxicity. The compounds of chromium(VI) pose serious dangers to biological systems, whereas those of chromium(III) are relatively non-toxic [3, 4]. On the other hand, chromium(VI) is one of the most versatile available oxidizing agents for the oxidation of organic and inorganic compounds. It can be reduced to lower oxidation states by various biological and chemical reductants. The chemistry of the intermediate oxidation states Cr(V) and Cr(IV) which observed during the reduction of chromium(VI) has been attracted many researchers due to their implication in the mechanism of Cr–induced cancers [5].
Although, various reports in the literature are available on the kinetics of chromium(VI) oxidation of various inorganic [6-10] and organic [11-23] compounds, no attention has been focused on the oxidation of polyamines by this oxidant. This leads us to investigate the kinetics of oxidation of cadaverine as a toxic diamine compound by chromium(VI) in different acidic media, namely perchloric and sulfuric acids. The objectives of this investigation were to establish the optimum conditions affecting the oxidation of such diamine substrate by chromium(VI), to examine the effect of acidic medium used and finally to elucidate a plausible oxidation mechanism.
2. Experimental
2.1. Materials
A stock solution of cadaverine was prepared by dissolving the sample (BDH) in double distilled water. A fresh solution of chromium(VI) oxidant was prepared before each experiment and it was standardized spectrophotometrically. All other chemicals used in the present study were reagent grade and their solutions were prepared by dissolving the requisite amounts of the samples in doubly distilled water.
2.2. Kinetic Measurements
In the present study, the reactants were formulated such that the oxidation reactions were of pseudo-first order kinetics where the cadaverine reductant was present in a large excess relative to chromium(VI) oxidant. The ionic strengths of the reactions media were maintained constant by addition of sodium perchlorate in case of perchloric acid medium and sodium sulfate in sulfuric acid medium. The reactions temperature (25 °C) was controlled within ±0.1 °C. The rates of oxidation reactions were followed by tracing the decay in chromium(VI) absorbance at its maximum absorption wavelength, λmax = 348 nm. The absorption measurements were made in a thermostatted Shimadzu UV-VIS-NIR-3600 double-beam spectrophotometer.
The observed-first order rate constants were determined as the gradients of ln(absorbance) versus time plots. Average values of at least two independent kinetic runs of the rate constants were taken for the analysis. The kinetic runs were reproducible to within ±3%. Few kinetic runs were carried out after bubbling purified nitrogen and compared with those taken under air, and the results were found to be the same. Thus the dissolved oxygen does not have any effect on the rate constant.
3. Result
3.1. Spectral Changes
The spectral changes throughout oxidation of cadaverine by chromium(VI) in both perchloric and sulfuric acids media are shown in Figure 1 (a) and (b), respectively. In both cases, the scanned spectra indicate gradual decay of chromium(VI) band at its absorption maximum with time as a result of its reduction by the cadaverine substrate. It can be observed that the rate of decay of chromium(VI) band in sulfuric acid medium is higher than that observed in perchloric acid medium.
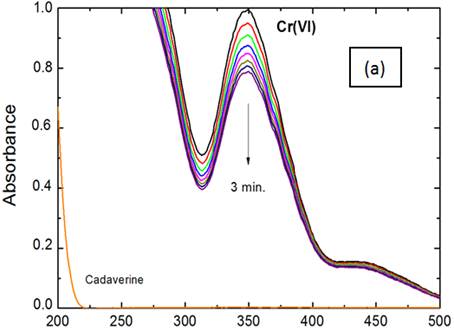
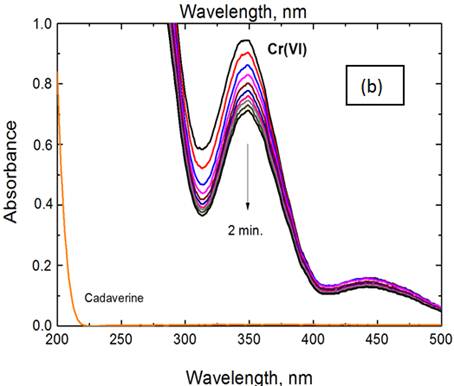
Figure 1. Spectral changes throughout chromium(VI) oxidations of cadaverine in: (a) perchloric and, (b) sulfuric acids media. [Cr(VI)] = 5.0 x 10-4, [CAD] = 6.0 x 10-3, [H+] = 1.0 and I = 2.0 mol dm-3 at 25°C.
3.2. Reactions Stoichiometry and Products Analysis
The stoichiometry of the oxidation reactions were determined spectrophotometrically at λ = 348 nm at a constant [H+] = 1.0 mol dm-3 and ionic strength of 2.0 mol dm-3, indicated consumption of two moles of chromium(VI) per three moles of cadaverine reductant to yield the final oxidation products as shown in the following equation,
3 H2N(CH2)5NH2 + 2 HCrO4- + H2O → 3 H2N(CH2)4CHO + 3 NH3 + 2 H2CrO3- (1)
where H2N(CH2)5NH2 and H2N(CH2)4CHO are cadaverine and its corresponding aldehyde (5-aminopentanal), respectively. The product aldehyde was identified by liquid chromatography (HPLC) and by spot test [24], and was also estimated quantitatively as its 2,4-dinitrophenylhydrazone derivative [25], and ammonia was identified by Nessler’s reagent [25].
3.3. Reaction Orders
The orders of the oxidation reactions with respect to the reactants were determined from the slopes of log kobs versus log(concentration) plots by varying the concentrations of cadaverine and perchloric acid or sulfuric acid, in turn, while keeping other conditions constant.
The concentration chromium(VI) oxidant was changed in the range of (1.0 - 8.0) x 10-4 mol dm-3 at constant concentrations of all other reagents. Plots of ln(absorbance) versus time were found to be linear for more than two half-lives of the reactions. This linearity indicated that the reactions were first order with respect to chromium(VI) concentration. The first-order dependence was also confirmed by the independence of the first order rate constants (kobs) on the different initial concentrations of chromium(VI) as listed in Table 1.
In both perchloric and sulfuric acids media, the effect of cadaverine concentrations was examined by measuring the values of kobs at various concentrations of cadaverine at constant [Cr(VI)], [H+], ionic strength and temperature. The results showed that increasing the concentration of cadaverine was found to increase the oxidation rates. The plots of kobs versus [CAD] were linear with positive intercepts (Figure 2) confirming that the reactions order with respect to [CAD] was less than unity.
To examine the effect of hydrogen ion concentration on the oxidation rates, kinetic runs were carried out at various hydrogen ion concentrations while other variables were kept constant. The results indicated that increase [H+] increased the oxidation rates (Table 1). The reactions order with respect to [H+] was found to be fractional-second which was confirmed as the gradients of the plots of log kobs versus log [H+] as shown in Figure 3.
3.4. Effect of Ionic Strength and Dielectric Constant
The ionic strength was varied from 2.0 to 4.0mol dm-3 at constant [CAD], [Cr(VI)] and [H+]. Increasing the ionic strength had a negligible effect on the oxidation rates (Table 1). Furthermore, the effect of the dielectric constant (D) of the reactions media on the oxidation rates has been investigated at different solvent compositions of acetic acid and water in the reactions media. The results showed that variation of dielectric constant of the media did not affect significantly the oxidation rates.
3.5. Effect of [Mn(II)]
The involvement of chromium(IV) as an intermediate species of chromium in the oxidation reactions was investigated by addition of various concentrations of Mn(II) ion to the reaction media. The results showed that the oxidation rates decreased with increasing [Mn(II)] as illustrated in Figure 4.
Table 1. Effect of variation of [Cr(VI)], [CAD], [H+] and ionic strength (I) on the observed-first order rate constant kobs in the oxidation of cadaverine by chromium(VI) in perchloric and sulfuric acids media at 25°C.
104 [Cr(VI)] (mol dm-3) | 103 [CAD] (mol dm-3) | [H+] (mol dm-3) | I (mol dm-3) | 105 kobs (s-1) |
Perchloric | Sulfuric |
2.0 | 6.0 | 1.0 | 2.0 | 26.3 | 37.1 |
3.0 | 6.0 | 1.0 | 2.0 | 25.9 | 38.7 |
4.0 | 6.0 | 1.0 | 2.0 | 27.2 | 39.0 |
5.0 | 6.0 | 1.0 | 2.0 | 26.4 | 37.6 |
6.0 | 6.0 | 1.0 | 2.0 | 28.0 | 36.2 |
8.0 | 6.0 | 1.0 | 2.0 | 25.6 | 37.0 |
5.0 | 2.0 | 1.0 | 2.0 | 9.9 | 14.1 |
5.0 | 4.0 | 1.0 | 2.0 | 18.2 | 26.3 |
5.0 | 6.0 | 1.0 | 2.0 | 26.4 | 37.6 |
5.0 | 8.0 | 1.0 | 2.0 | 29.8 | 49.0 |
5.0 | 10.0 | 1.0 | 2.0 | 38.1 | 60.1 |
5.0 | 12.0 | 1.0 | 2.0 | 45.0 | 69.6 |
5.0 | 6.0 | 0.4 | 2.0 | 4.4 | 5.8 |
5.0 | 6.0 | 0.7 | 2.0 | 14.8 | 19.6 |
5.0 | 6.0 | 1.0 | 2.0 | 26.4 | 37.6 |
5.0 | 6.0 | 1.3 | 2.0 | 42.4 | 49.8 |
5.0 | 6.0 | 1.6 | 2.0 | 59.1 | 66.3 |
5.0 | 6.0 | 1.9 | 2.0 | 78.0 | 85.1 |
5.0 | 6.0 | 1.0 | 2.0 | 26.4 | 37.6 |
5.0 | 6.0 | 1.0 | 2.3 | 23.8 | 40.1 |
5.0 | 6.0 | 1.0 | 2.6 | 25.7 | 38.9 |
5.0 | 6.0 | 1.0 | 3.0 | 27.6 | 39.8 |
5.0 | 6.0 | 1.0 | 3.5 | 26.3 | 38.4 |
5.0 | 6.0 | 1.0 | 4.0 | 27.1 | 36.5 |
Experimental error ± 3%
3.6. Effect of Temperature
The oxidation reactions in both acids were investigated at five different temperatures in the range of 15 – 35°C. The oxidation rates were found to increase with raising temperature. The activation parameters of the second order rate constants (k2) were calculated using Arrhenius and Eyring plots and were listed in Table 2.
Table 2. Activation parameters of the second order rate constants k2 in the oxidation of cadaverine by chromium(VI) in perchloric and sulfuric acids media. [CAD] = 6.0 x 10-3, [Cr(VI)] = 5.0 x 10-5, [H+] = 1.0 and I = 2.0 mol dm-3.
Acid | DS≠, J mol-1K-1 | DH≠, kJ mol-1 | DG≠298, kJ mol-1 | Ea≠, kJ mol-1 |
Perchloric | -77.98 | 47.12 | 70.59 | 50.04 |
Sulfuric | -68.21 | 38.90 | 59.20 | 41.02 |
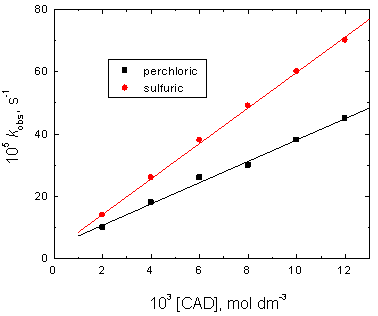
Figure 2. Effect of [CAD] on the observed-first order rate constants kobs in the oxidation of cadaverine by chromium(VI) in perchloric and sulfuric acids media. [Cr(VI)] = 5.0 x 10-5, [H+] = 1.0 and I = 2.0 mol dm-3 at 25°C.
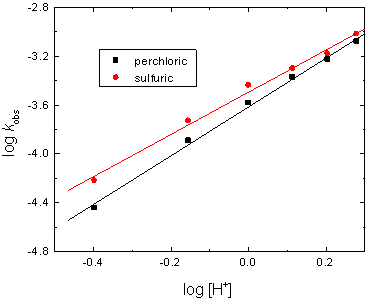
Figure 3. Plots of log kobs versus log [H+] in the oxidation of cadaverine by chromium(VI) in perchloric and sulfuric acids media. [CAD] = 6.0 x 10-3, [Cr(VI)] = 5.0 x 10-5 and I = 2.0 mol dm-3 at 25°C.
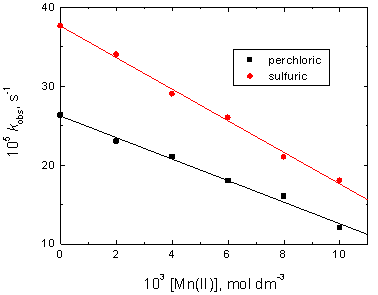
Figure 4. Effect of manganese(II) on the observed-first order rate constants kobs in the oxidation of cadaverine by chromium(VI) in perchloric and sulfuric acids media. [CAD] = 6.0 x 10-3, [Cr(VI)] = 5.0 x 10-5, [H+] = 1.0 and I = 2.0 mol dm-3 at 25°C.
3.7. Polymerization Test
Intervention of free radicals during the oxidation reactions in both acids was examined by the polymerization test where known quantities of acrylonitrile monomer was added to the partially oxidized reactions mixtures and kept for about 6 h in an inert atmosphere (N2). On diluting the mixtures with methanol, no white precipitates were formed thus confirming the absence of free radicals in the oxidation reactions.
4. Discussion
There are two suggested mechanisms for electron transfer in chromium(VI) redox reactions, the first suggested mechanism involve a successive one-electron transfer in two steps and the second one was a simultaneous two-electron transfer in a single step [26]. Both mechanisms may be considered during reduction of Cr(VI) to form either Cr(V) or Cr(IV) species, respectively [27, 28]. In the present study, the negative result of free radical test excluded formation of Cr(V) species. Furthermore, the involvement of Cr(IV) species in the oxidation reactions was confirmed from the decrease in the oxidation rates upon addition of manganese(II) to the reactions media (Figure 4). This is because Mn(II) has been recognized as a frequently tool for trapping Cr(IV) intermediate species [29] according to the equation, Cr(IV) + Mn(II) = Cr(III) + Mn(III). On the other hand, chromium(VI) in acidic media was suggested [30, 31] to exist mainly as acid chromate, H2CrO4, as represented by the first step in Scheme 1.
The present reactions between chromium(VI) and cadaverine in both perchloric and sulfuric acids media showed a stoichiometry of 2: 3 (Cr(VI): CAD) with a first order dependence with respect to [Cr(VI)], less than unit order dependences on [CAD], and fractional-second order dependences on [H+]. The obtained fractional-second order dependences on [H+] was explained by protonation of both chromium(VI) oxidant and cadaverine substrate to form more reactive species of both, i.e., H2CrO4 and CAD+. The less than unit order dependences with respect to cadaverine concentration was attributed to complexes(C) formation between the reactive species of both cadaverine and chromium(VI) in both acids media in prior to the rate-determining step. The formation of the complexes was also proved kinetically [32] by the obtained positive intercepts of the plots of 1/kobs versus 1/[CAD] as shown in Figure 5. Complexes formation between chromium(VI) and different organic compounds in acidic media was also reported by Fawzy et. al [11-17, 33, 34]. Furthermore, the negligible effects of both ionic strength and dielectric constant of the reactions media were consistent with reactions between two neutral molecules or between neutral molecule and an ion [35, 36] i.e. between H2CrO4 and CAD+
Based on the forgoing aspects, the most reasonable reaction mechanism which may be suggested (see Scheme I), involves protonation of both chromium(VI) oxidant (step I) and cadaverine substrate (step II), followed by a fast complexation between the kinetically active species of chromium(VI) (H2CrO4) and cadaverine (CAD+), step (III), forming intermediate complexes (C). The latter was slowly decomposed in the rate-determining step followed by subsequent fast steps, (IV) - (VII), to give rise to the final oxidation products.
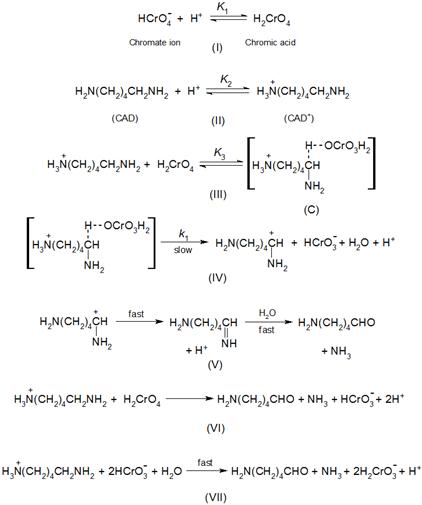
Scheme 1. Mechanistic scheme of the oxidation of cadaverine by chromium(VI) in acidic media.
According to the suggested mechanistic Scheme 1, the relationship between the oxidation rates and oxidant, reductant and hydrogen ion concentrations was represented by the following rate-law expression,
(2)
Under pseudo-first order condition,
(3)
Comparison of Eqs. (2) and (3) leads to,
(4)
(5)
In view of Eq. (5), plots of 1/kobs versus 1/[CAD] at constant [H+], and 1/kobs versus 1/[H+]2 at constant [CAD] should be linear with positive intercepts as were obtained experimentally as illustrated in Figures 5 and 6, respectively. This behavior confirms the suggested mechanism.
On the other hand, the obtained large negative values of DS≠ are consistent with the formation of compacted intermediate complexes [37, 38]. The experimental values of DH≠ and DS≠ were both favorable for electron-transfer process [44]. Again, the positive values of DH≠ and DG≠ indicate endothermic formation of the intermediate complexes and their non-spontaneities, respectively.
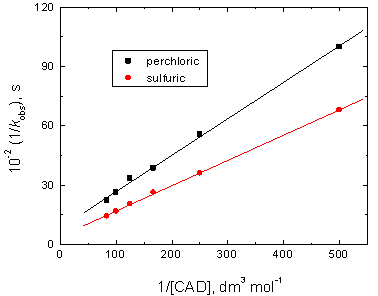
Figure 5. Plots of 1/kobs versus 1/[CAD] in the oxidation of cadaverine by chromium(VI) in perchloric and sulfuric acids media. [Cr(VI)] = 5.0 x10-5, [H+] = 1.0 and I = 2.0 mol dm-3 at 25°C.
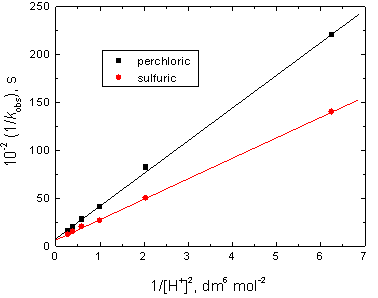
Figure 6. Plots of 1/kobs versus 1/[H+]2 in the oxidation of cadaverine by chromium(VI) in perchloric and sulfuric acids media. [CAD] = 6.0 x 10-3, [Cr(VI)] = 5.0 x 10-5and I = 2.0 mol dm-3 at 25°C.
5. Conclusions
Chromium(VI) oxidation of cadaverine in both perchloric and sulfuric acids media was studied. In both acids In both acids, the final oxidation products of cadaverine were identified as 5-aminopentanal and ammonia. Under comparable experimental conditions, the oxidation rate of cadaverine in sulfuric acid was found to be higher than that in perchloric acid. The plausible oxidation mechanism were proposed and the rate-law expression was derived. The activation parameters with respect to the second order rate constants were evaluated.
References
- Lewis, Robert Alan (1998). Lewis' Dictionary of Toxicology. CRC Press, pp. 212.
- Yeoman CJ, et al (2013). A multi-omic systems-based approach reveals metabolic markers of bacterial vaginosis and insight into the disease, PloS one 8: e56111.
- Katz SA, Salem H (1993) The toxicology of chromium with respect to its chemical speciation: a review. J Appl Toxicol.13: 217–224.
- Kostecki PT (1998) Chromium in soil: perspectives in chemistry, health, and environmental regulation. J. Soil. Contamin. 6: 561–568.
- Costa M (1997) Toxicity and carcinogenicity of CrVI in animal models and humans. CRC, Crit. Rev. Toxicol. 27: 431-442.
- Chimatadar SA, Koujalagi SB, Nandibewoor ST (2001) Kinetics and mechanism of palladium(II) catalyzed chromium(VI) oxidation of mercury(I) in aqueous sulphuric acid,Transition Met. Chem.26: 662-667.
- Chimatadar SA, Basavaraj T, Nandibewoor ST (2006) Mechanistic study of quinoliniumdichromate (QDC) oxidation of mercury(I) in aqueous sulfuric acid in the presence of micro amounts of palladium(II). Autocatalysis in catalysis.Polyhedron25: 2976-2984.
- Sen Gupta KK, Chakladar JK (1974) Kinetics of the chromic acid oxidation of arsenic(II). J. Chem. Soc. Dalton Trans. 2: 222–225.
- Sen Gupta KK, Chakladar JK, Chatterjee AK, Chakladar JK (1973) Kinetics of the oxidation of hypophosphorous and phosphorous acids by chromium(VI). J. Inorg. Nucl. Chem. 35: 901–908.
- Espenson JH (1970) Oxidation of transition metal complexes by chromium(VI). Accounts Chem. Res. 3: 347–351.
- Fawzy A, Ashour SS, Musleh MA, Hassan RM, Asghar BH (2016) Kinetics and mechanistic approach to the chromic acid oxidation of L-tryptophan with a spectral detection of chromium(III) product. J. Saudi Chem. Soc.20: 450-458.
- Fawzy A, Althagafi I, Tirkistani F, Shaaban M, Morad M (2016) Chromic acid oxidation of methylaminopyrazole formamidine in sulfuric acid medium: a kinetic and mechanistic approach. Am. J. Phys. Chem. 5: 1-9.
- Fawzy A, Altass HM (2016) Ruthenium(III)-catalyzed oxidation of alginate and pectate biopolymers by chromic acid in aqueous perchlorate solutions: a comparative kinetic study. Transition Met. Chem. 41: 115-124.
- Hassan RM, Ahmed SM, Fawzy A, Abdel-Kader DA, Ikeda Y, Takagi HD (2010) Acid-catalyzed oxidation of carboxymethyl cellulose polysaccharide by chromic acid in aqueous perchlorate solutions. a kinetics study. Cat. Commun., 11: 611-615.
- Fawzy A, Althagafi I, Khairou K, Hassan R, Yarkandi N, Almazroai L, Bawazeer T (2016) Kinetics and mechanistic aspects of oxidation of iota- and lambda-carrageenans by chromium(VI) in aqueous perchlorate solutions.Chem. Sci. Rev. Lett.,4: 1293-1304.
- Fawzy A, Zaafarany IA, Khairou KS, Althagafi I, AlfahemiJ (2016) Kinetics and mechanism of oxidation of vanillin by chromium(VI) in sulfuric acid solutions. Modern Chem. Appl., 4: 179-185.
- Fawzy A, Zaafarany IA, Althagafi II, Altass HM, Morad MH, Khairou KS (2016) Kinetics and mechanistic approach to the chromic acid oxidative degradation of atropine drug in perchlorate solutions and the effect of ruthenium(III) catalyst.Modern Chem. Appl., in press.
- Hasan F, Rocek J (1975) Three-electron oxidations. IX. Chromic acid oxidation of glycolic acid. J. Am. Chem. Soc. 97: 1444–1450.
- Khan Z, Id-Din K (2001) Effect of manganese(II) ions on the oxidation of maleic and oxaloethanoic acids by aqueous H2CrO4. Transition Met. Chem. 26: 672–678.
- Manhas MS, Kumar P, Mohamed F, Khan Z (2008) Oxidative degradation of non-ionic surfactant (Triton X-100) by chromium(VI). Coll. Surf. A 320: 240–246.
- Odebunmi EO, Obike AI, Owalude SO (2009) Kinetics and mechanism of oxidation of D-xylose and L-arabinose by chromium(VI) ions in perchloric acid medium. Int. J. Biolog. Chem. Sci. 3: 178-185.
- Bayen R, Das AK (2009) Kinetics and mechanism of oxidation of D-galactose by chromium(VI) in presence of 2,2´-bipyridine catalyst in aqueous micellar media. Open Catal.J. 2: 71-78.
- Sen Gupta KK, Sarkar T (1975) Kinetics of the chromic acid oxidation of glyoxylic and pyruvic acids. Tetrahedron 31: 123–129.
- Feigl F (1957) Spot Tests in Organic Analysis, Elsevier, New York, NY, USA.
- Vogel AI (1973) Text Book of Practical Organic Chemistry including Quantitative Organic Analysis. 3rd edn, pp 332, ELBS, Longman.
- Espenson JH, King EL (1963) Kinetics and mechanism of reaction of chromium(VI) and iron(II) species in acidic medium.J. Am. Chem. Soc.85: 3328–3333.
- Espenson EH, Wang RT (1972) The oxidation of uranium(IV) by chromium(VI) and the induced oxidation of iodide ions. Inorg. Chem. 11: 955–959.
- Bose RN, Moghaddas B, Gelerinter E (1992) Long-lived chromium(IV) and chromium(V) metabolites in the chromium(VI)-glutathione reaction: NMR, ESR, HPLC, and kinetic characterization. Inorg. Chem. 31: 1987-1994.
- Milazzo G, Caroli S, Sharma VK (1978) Tables of Standard Electrode Metal Potentials, Wiley & Sons, New York.
- Bailey N, Carrington A, Lott KAK, Symons MCR (1960) Structure and reactivity of the oxyanions of transition metals. Part VIII. Acidities and spectra of protonated oxyanions. J. Chem. Soc. 290–297.
- Sasaki Y (1962) Equilibrium studies on polyanions. 9. The first steps of acidification of chromate Ion in 3 M Na(ClO4) Medium at 25 degrees C. Acta Chem. Scand.16: 719–734.
- Michaelis L, Menten ML (1913) The kinetics of invertase action. Biochem. Z 49: 333–369.
- Naik PK, Chimatadar SA, Nandibewoor ST (2008) A kinetic and mechanistic study of the oxidation of tyrosine by chromium(VI) in aqueous perchloric acid medium. Transition Met. Chem. 33: 405–410.
- Day MC, Selbin JJ (1964) Theoretical inorganic chemistry, Reinhold Publishing Corporation, New York.
- Frost AA, Person RG (1971) Kinetics and Mechanism, Wiley Eastern, New Delhi, 1970; C. H. Rochester, Progress in Reaction Kinetics, Pergamon Press, Oxford; Laidler K (1965) Chemical Kinetics, McGraw-Hill, New York.
- Amis ES (1966) Solvent Effects on Reaction Rates and Mechanism, Academic Press, New York.
- Hicks KW, Toppen DL, Linck RG (1972) Inner-sphere electron-transfer reactions of vanadium(II) with azidoamine complexes of cobalt(III). Inorg. Chem.11: 310-315.
- Weissberger A (1974) In Investigation of Rates and Mechanism of Reactions in Techniques of Chemistry, John Wiley & Sons,pp. 421.