Synthesis of Na-zeolite on the Basis of Dual Cation/Anion Exchange Capacity with Regeneration Capabilities
John Mugisa1, *, G. W. Nyakairu2, Farad Sagala3
1Faculty of Engineering, International University of East Africa, Kampala, Uganda
2Department of Chemistry, Makerere University, Kampala, Uganda
3Department of Petroleum Engineering, Universiti Teknologi, Johor Bahru, Malaysia
Email address

(J. Mugisa)
*Corresponding author
Citation
John Mugisa, G. W. Nyakairu, Farad Sagala. Synthesis of Na-zeolite on the Basis of Dual Cation/Anion Exchange Capacity with Regeneration Capabilities. Journal of Materials Sciences and Applications. Vol. 2, No. 3, 2016, pp. 20-24.
Abstract
Zeolites are aluminosilicate solids bearing a negatively charged honeycomb framework of micro pores into which molecules may be adsorbed for separation purposes as well as catalysis of chemical reactions. In this study, Na-Zeolite was synthesized via a hydrothermal reaction on the basis of dual cation/anion exchange capacities with regeneration capabilities. The sodium silicate solution used in the laboratory experiments was in the concentration range of 62-65%. Both anionic and cationic functional groups together are derived from the concentrated sodium silicate solution where during depolymerization of siloxane bonds takes place not only into silanol anions but also silanol cations. The silanol cations and anions polymerize into cyclic anionic and cationic functional groups respectively showing dual cation and anion exchange property. X-ray diffraction pattern indicates a crystalline structure with the material present in one phase. Cation exchange capacity (CEC) and anion exchange capacity (AEC) capacities derived were 26.9 and 28.4 respectively with the produced zeolites being thermally stable at 250°C. Regeneration of the spent zeolite was achieved by treating with 1.05N HCl. Summing up the results, we can show that zeolite formation with dual ion exchange sites is possible by hydrothermal reaction. Besides the importance for separation chemistry, the reaction via this method is of interest for zeolite chemistry as the re-use of the spent zeolite is possible under economically conditions of low energy consumption and short reaction periods.
Keywords
Na-zeolites, Dual Exchange Capacity, Hydrothermal, Regeneration
1. Introduction
Zeolites are aluminosilicates that occur both as natural and as produced synthetic [1-3]with micro porous crystalline frameworks built of tetrahedral units of silica (SiO4) and alumina (AlO4) which arrange to form a network of interconnected pores and channels of molecular dimensions [4, 5].
Zeolites are very important materials because of their wide industrial applications such as sorption, ion exchange and catalysis [2, 3]. In recent years, many investigations have been on the development of low-cost and effective synthesis alternatives [3] for products with superior capabilities [5-8].
Among the methods available for synthesis, hydrothermal approach is most preferred because it is simple, fast, cheap, produces high yield and can easily be scaled [2, 9] to produce ideal zeolitic material with special morphology. As one of the wide application, synthetic zeolites have extensively been applied in cationic species removal [1, 10, 11]; however, few investigations have been made on the anionic species removal from aqueous effluents. According to [2, 3, 5], zeolites need to be functionalized to promote their capacity to uptake anionic species. In addition, the aging and the regeneration of the exhausted material needs to be studied [12]. The regeneration of the spent adsorbent enables to increase the adsorbent middle life and contributes notably to reduce the cost of adsorption process. In this brief report, a simple and novel approach to synthesize faceted, micro-crystalline silicate based dual cation-/anion exchange zeolites with regeneration capabilities is reported. This method is primarily based upon zeolite synthesis using concentrated sodium silicate solution to impart special functionalities to the produced zeolite material.
2. Materials and Methods
This article is devoted to the study of the process for preparing silica based dual ion exchange zeolites on the same crystalline structure with high exchange capacity using sodium silicate solution with concentration range of 62-65% (Na2O·1.7SiO2). The sodium silicate solution was purchased from Kenya Industrial research and development Institute (KIRDI).
2.1. Synthesis Procedure
Silica based dual exchange zeolites was synthesized by adding 28ml of conc. H2SO4 to sodium silicate solution (120ml) in a beaker at a time and mixed sufficiently well for 30min. for the complete precipitation of zeolite at pH=7.0.About 50% of the Na2SO4 formed in the reaction gets adsorbed on to the zeolite ppt. and for its complete removal, the zeolite ppt. was kept under 200ml of hot water at 80-90°C for 2 hrs. This was then transferred to a glass column and washed free of (SO42-) ions with deionized H2O. The zeolite obtained was in (H+) and (Na+) forms in the ratio of 1.0: 0.20 as cation exchangeable ions and (OH-) as anion–exchangeable ions. The zeolite was converted to (H+, OH-) form by treating with 14.0ml of 3.171N HCl and washing with deionized H2O, followed by drying in an oven at 120°C for 3-4 hours.
2.2. Sample Characterization
An x-ray diffraction study on the produced zeolite was carried out using SHIMADZO X-ray diffractometer, XD-D1.
2.3. Adsorption and Regeneration Experiments
Sorption experiments were carried using 2.0g sample of dried zeolite that was treated with 8.2ml of 46.56% CaCl2 solution (containing 1376mg of Ca2+ and 2442mg of Cl- ions) and mixed for 2.0 min. This was followed by filtration and washing with d.H2O. The filtrate and washings were 50ml analyzed for un-exchanged (Ca2+) and (Cl-) ions. The spent zeolite was regenerated by treating with 150ml of 1.05N HCl. The volume of the filtrate was analyzed for eluted Ca2+ and Cl- ions and un-exchanged HCl.
2.4. Thermostability Studies
Thermostability experiments were done using 25.0g of dual ion exchange zeolite in (H+, OH-) form containing a total of 660.0meq as CEC and 710meq as AEC that was dried at 250°C for 4 hours. It was wetted sufficiently with de ionized water packed in a glass column and treated with 650ml of 46.56% CaCl2, containing 17116.0mg Ca2+ and 30376 Cl- ions solution at the flow rate of 2.0ml per minute for the simultaneous exchange of (H+) and (OH-) ions on the zeolite with (Ca2+) and (Cl-)ions respectively.
3. Results and Discussion
Table 1. Adsorption results for Ca2+ ions exchanged with H+ ions.
Calculations | Concentration in Milligrams (mg) |
Ca2+ used for exchange | 1376 |
Ca2+ un-exchanged | 300 |
Ca2+ exchanged | 1076 |
Milli-equivalent (mEq) | 53.8 |
Cation Exchange Capacity (CEC)/g | 26.9 |
Formula: meq= mg÷ equiv. wt.
Table 2. Adsorption results for (Cl-) ions exchanged with (OH-) ions.
Calculations | Concentration in Milligrams (mg) |
Cl- used for exchange | 2442 |
Cl- un-exchanged | 425 |
Cl- exchanged | 2017 |
Milli-equivalent (mEq) | 56.8 |
Anion Exchange Capacity (AEC)/g | 28.4 |
Formula: meq= mg÷ equiv. wt.
Observed experimental results in Table 1 show that, of the total 1376 mg Ca2+ ions used for cation exchange, only 300mg passed through the zeolite loaded column un-exchanged, reflecting the maximum removal efficiency of 78% (26.9 CEC/g). Similarly as indicated by Table 2, out of 2442 mg (Cl-) ions used for anionic exchange, 425 mg (Cl-) ions eluted out of the column un-exchanged indicating sorption efficiency of 82% (AEC/g 28.4 /g). The practical activity of the zeolite realized can be can be related to the created active sites during synthesis via this method. This agrees with the conclusion by [13-15], that a combination of factors including shapes and sizes of the crystals, channels and pores are critical for a zeolite’s chemical reactivity. During the ion-exchange process, metal ions move through the pores of the zeolite mass through channels of the lattice as they replace exchangeable ions (mainly sodium and hydroxyl ions). Diffusion is faster through the pores and is retarded when the ions move through the smaller diameter channels [16].
Table 3. Simultaneous cation and anion exchange reactions during recycling dual ion exchanger zeolite.
No. of cycles | CEC(Meq/g) | AEC (Meq/g) | Zeolite Form | Cycle |
I | 26.90 | 28.40 | (H+, OH-) | Exhaustion |
| 26.90 | 28.80 | (Ca2+, Cl-) | Regeneration |
II | 26.30 | 27.80 | (H+, OH-) | Exhaustion |
| 26.90 | 28.50 | (Ca2+, Cl-) | Regeneration |
III | 26.50 | 28.20 | (H+, OH-) | Exhaustion |
| 26.30 | 27.90 | (Ca2+, Cl-) | Regeneration |
The Na-zeolite showed potential for better performance on Ca2+ and Cl- ions adsorption. After accelerated aging with 15% CaCl2, the exhausted zeolite was regenerated in acidic media and the resultant material was re-tested to evaluate its regeneration capacity without substituting the zeolite in any cycle.As shown in Table 3 the regenerated zeolite exhibited the original adsorption capacity in the observed three cycles. The effectiveness of acid treatment is said to be dependent on the chemical composition, structure, mineral purity, and the working conditions. Remediation of the exhausted zeolite ions with H+ and OH- ions is significantly dependent on the solution acidity and cation hydration enthalpy [12, 17]. Hydrochloric acid solution treatment leads to decationization and deanionization, obtaining the so-called "hydrogen/hydroxyl form" zeolites [12, 18].
3.1. Thermostability Results
The weight of the zeolite after drying was 23.50g. The reduction in weight by 6.0% at 250°C was due to evaporation of water molecules present in the cavity of the zeolite [9]. However both the cation and anion exchange capacities present initially remained unaltered at this temperature confirming the thermal stability of the zeolite. Such property (thermal stability) is important for the synthesized zeolite which is used as a sorbent and for catalysis at elevated temperatures [15].
3.2. Dual Ion Exchange Property
Previous studies have reported sodium silicate solution to contain only silanol anions [7, 19], however during this study, a surprise and very interesting observation made was that when concentrated sodium silicate solution was used, silanol cations were formed along with silanol anions. According to [17], impregnation solutions of cations or cationic complexes can also be used in circumstances where there is low ion exchange capacity in the zeolite being used.In this case the metal distributes itself both inside the zeolite and on the binder. The existence of silanol cations and anions can be explained by depolymerization reaction of siloxane bond; Si─O─Si (in presence of Na+, O2─, and OH─ ions) which gives breakdown products that not only include silanol anions (Si─O─) but also silanol cations (Si─OH+) together as shown by equation 1.
(1)
The silanol species undergo polymerization in the presence of catalytic influence of mineral acids into polyanionic and polycationic functional groups respectively as exchange sites for cations and anions. These groups enter the crystalline structures at the non-bridging O atoms during the synthesis imparting zeolite with dual cation-/anion exchange properties. During neutralization reaction for synthesis (see equation 2);
(2)
The dissociation of water molecules that occur in presence of heat of neutralization act as a constraint on equilibrium and shifts it towards dissociation of water molecules into (H+) and (OH-) ions according to Le-Chatelier van’t Hoff’s theorem as shown by equation 3.
(3)
The dissociated (H+) and (OH-) ions due to their ion-hydration and electrostatic ion-bonding energies for "ion pairs" get embedded with polyanionic and polycationic functional groups present on the zeolite structure result in dual cation-/anion exchange sites on the produced Na-zeolites as shown by Figures 1 and 2.
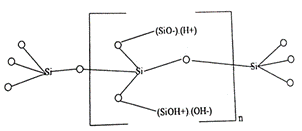
Figure 1. Crystalline metasilicate having anionic (SiO─), and cationic (SiOH+) functional groups with respective exchangeable cations (H+) and anions (OH─).
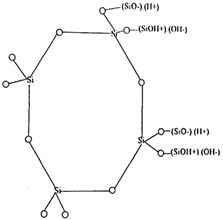
Figure 2. Crystalline ring structure having cationic (SiOH+) and anionic (SiO─) functional groups with respective exchangeable cations (H+) and anions (OH─).
X-ray diffraction pattern of the produced zeolite is represented in Figure 3, manifests that the synthesized zeolite material was present in one phase with highly crystalline structure. The observed pattern of the diffractogram matched the main peaks of Na-zeolites at 2Ө values of 20.9o, 23.1o, 24.9o, 27.1o, 29.4o, 31.7o, 32.7o, 35.9o, 39.4o, 43.1o, 43.8o, 45.9o, 47.5o, 49.9o, 55.6o that were reported by[20].
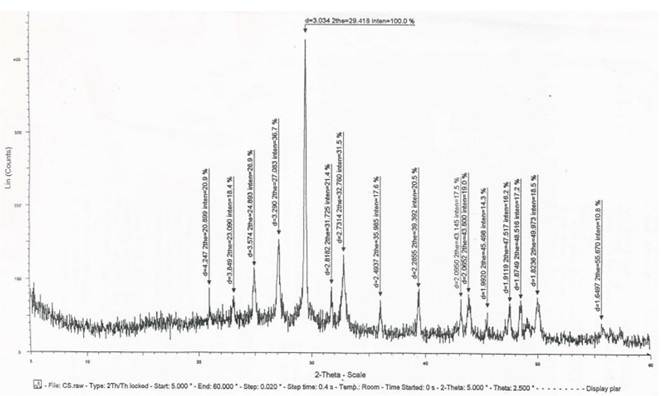
Figure 3. X-ray diffractogram of crystalline silica (SiO2) dual ion exchange zeolite in (H+) and (OH-) forms with CEC 26.5 and AEC 28.5meq/g.
4. Conclusion
In this study, a surprise and very interesting observation was made when a zeolite was prepared from concentrated sodium silicate solution by the newly developed method which showed both cation and anion exchange properties. This was due to the presence of both cationic and anionic functional groups together in the interstitial network of the zeolite being derived from the concentrated solution of sodium silicate. Depolymerization of siloxane bonds takes place into silanol anions and cations. The silanol cations and anions polymerize into cyclic anionic and cationic functional groups respectively showing dual cation and anion exchange property. Studies also indicate a thermally stable zeolite at 250°C. The spent zeolite can be regenerated by treating with HCl.
Acknowledgement
The authors acknowledge International University of East Africa for funding and Uganda Industrial Research Institute Chemistry Laboratories for the facilities given to carry out the analytical work.
References
- Petit, Y. and W. Rond, Linde Type a Zeolite and Type Y Faujasite as a Solid-Phase for Lead, Cadmium, Nickel and Cobalt Preconcentration and Determination Using a Flow Injection System Coupled to Flame Atomic Absorption Spectrometry. American Journal of Analytical Chemistry, 2013. Vol. 04No. 08: p. 11.
- Samedov, K. R. and U. A. Mammadova, Synthesis of Zeolitic Material on the Basis of Natural Raw Material Volcanic Ash in Presence of Tetraethylammonium Iodide. Advances in Chemical Engineering and Science, 2016. Vol. 06No. 01: p. 6.
- Hartmann, A., et al., Zeolite Synthesis under Insertion of Silica Rich Filtration Residues from Industrial Wastewater Reconditioning. Advances in Chemical Engineering and Science, 2014. Vol. 04No. 02: p. 15.
- Azizi, S. N., A. R. Samadi-Maybodi, and M. Yarmohammadi, Synthesis of NaA zeolite using PTMAOH (phenyltrimethylammoniumhydroxide): hydrothermal and microwave heating methods and comparison of their XRD patterns. Open Journal of Inorganic Chemistry, 2012. Vol. 02No. 01: p. 5.
- Mohamed, E. A., et al., Modeling and Optimizations of Phosphate Removal from Aqueous Solutions Using Synthetic Zeolite Na-A. Journal of Materials Science and Chemical Engineering, 2015. Vol. 03No. 09: p. 15.
- Guaya, D., et al., Simultaneous phosphate and ammonium removal from aqueous solution by a hydrated aluminum oxide modified natural zeolite. Chemical Engineering Journal, 2015. 271: p. 204-213.
- Dülger, A. and E. Yılmaz, Effectiveness of modified zeolites as adsorbent materials for frying oils. European Journal of Lipid Science and Technology, 2013. 115(6): p. 668-675.
- Li, C., et al., Surfactant modified zeolite as adsorbent for removal of humic acid from water. Applied Clay Science, 2011. 52(4): p. 353-357.
- Abou-Mesalam, M. M., Hydrothermal Synthesis and Characterization of a Novel Zirconium Oxide and Its Application as an Ion Exchanger. Advances in Chemical Engineering and Science, 2011. Vol. 01No. 01: p. 6.
- Munthali, M. W., et al., Decrease in Cation Exchange Capacity of Zeolites at Neutral pH: Examples and Proposals of a Determination Method. Journal of Materials Science and Chemical Engineering, 2014. Vol. 02No. 08: p. 5.
- Foo, K. Y. and B. H. Hameed, The environmental applications of activated carbon/zeolite composite materials. Advances in Colloid and Interface Science, 2011. 162(1–2): p. 22-28.
- Ates, A. and C. Hardacre, The effect of various treatment conditions on natural zeolites: Ion exchange, acidic, thermal and steam treatments. Journal of Colloid and Interface Science, 2012. 372: p. 130-140.
- Adriana, M. R., et al., Kinetic and Thermodynamic Study of Arsenic (V) Adsorption on P and W Aluminum Functionalized Zeolites and Its Regeneration. Journal of Water Resource and Protection, 2013. Vol. 05No. 08: p. 10.
- Jumean, F., L. Pappalardo, and H. Khoury, Removal of Pb(II) from Aqueous Solutions by Zeolites, Porcelanite and Sands: Correlation of Morphology and Chemical Composition to Batch Removal Efficiency. American Journal of Analytical Chemistry, 2015. Vol. 06No. 04: p. 8.
- Moneim, M. A. and E. A. Ahmed, Synthesis of Faujasite from Egyptian Clays: Characterizations and Removal of Heavy Metals. Geomaterials, 2015. Vol. 05No. 02: p. 9.
- Erdem, E., N. Karapinar, and R. Donat, The removal of heavy metal cations by natural zeolites. J Colloid Interface Sci, 2004. 280(2): p. 309-14.
- Rozić, M., et al., Decationization and dealumination of clinoptilolite tuff andammonium exchange on acid-modified tuff. Colloid Interf. Sci., 2005. 284(1): p. 48-56.
- Tomić, S., et al., Removal of Mg from spring water using natural clinoptilolite. Clay minerals, 2012. 47(1): p. 81-92.
- Li, Z. and R. S. Bowman, Regeneration of surfactant-modified zeolite after saturation with chromate and perchloroethylene. Water Res, 2001. 35(1): p. 322-6.
- Treacy, M. M. J. and J. B. Higgins, Collection of Simulated XRD Powder Patterns for Zeolites. 2001, Amsterdam: Elsevier.