Torso Flexion and Head Rotation are Additive, but not Synergistic, in Their Impacts Upon Exhalation Performance
Zachary J. Ankney, Katalin Komjati, Paul M. Nealen*
Department of Biology, Indiana University of Pennsylvania, Indiana, PA, U.S.A
Email address

(P. M. Nealen)
*Corresponding author
Citation
Zachary J. Ankney, Katalin Komjati, Paul M. Nealen. Torso Flexion and Head Rotation are Additive, but not Synergistic, in Their Impacts Upon Exhalation Performance. Health Sciences Research. Vol. 3, No. 2, 2016, pp. 23-29.
Abstract
Study Aim: Patient position during care has significant implications for patient pulmonary performance, such that standard guidelines exhibit for consideration of bedded patient torso angle in order to ensure sufficient pulmonary function. It is known that both torso flexion and head rotation independently impair exhalation performance, but their combined effects have not been examined, despite the fact that bed-ridden subjects routinely experience both simultaneously. Methods: To assess the magnitude and form of interaction that torso angle and head rotation exert on patient pulmonary performance, we used spirometry to measure exhalation function in healthy young adults in combinations of torso and head positions designed to mimic the positioning of bedridden patients. Subject maximal values for both Forced Vital Capacity (FVC) and Forced Expiratory Volume (FEV1) at each position were scored relative to individual predicted values, and compared using ANOVA for both main and interactive positioning effects. Results: As expected, head rotation alone caused decrements in both subject FVC and FEV1, while changes in torso angle exhibited a small influence on subject FEV1 alone. No significant interaction between torso angle and head rotation was revealed, suggesting that the effects of head rotation were uniform across all torso angles examined. The combined effects of both torso flexion and head rotation closely matched those predicted by additive combination of their independent effects, suggesting that their interaction is linear, and non-synergistic. Conclusion: While torso flexion and head rotation exhibit only modest effects on pulmonary function in healthy subjects, their combined effects are additive, and mutually consistent. As such, attention must be paid to both torso flexion and head rotation during patient care for subjects whose ventilatory performance is most crucial.
Keywords
Spirometry, Patient Positioning, Head Rotation, Torso Angle, Forced Vital Capacity (FVC), Forced Expiratory Volume (FEV)
1. Introduction
Patient positioning during long-term hospital care must carefully balance patient comfort with physiological function and treatment needs [1]. In particular, patient positioning which compromises airflow raises the risk of pulmonary infections, known to occur in a high proportion of intensive care patients [2]. One of the most important and effective mechanisms of pulmonary infection prevention is patient self-management of pulmonary flow, as subjects with compromised airflow may not effectively clear their airways of infectious agents [3] and are thus at risk of pneumonia contraction [4].
The importance of body posture to pulmonary flow has been previously investigated, with multiple studies suggesting that head and torso position individually can influence pulmonary function. Carlo et al. (1989) showed that airflow resistance is influenced by head position, as infant airflow resistance increased when the head was flexed 45° away from a neutral position [5]. This is similar to the findings of Badr et al. (2002), who reported a reduction in expiratory flow due to subject downward head tilt [6] and in contrast to Melissinos and Mead (1977), who reported increased airflow with neck hyperextension [7]. Behrakis et al. (1983) tested subjects in both supine and sitting positions, and found nearly a 30% reduction in resistance to pulmonary flow in the sitting position compared to the supine position [8], a result similar to that reported by Attinger et al. (1956) [9]. Gudmundsson et al. (1997) found that the body positions of standing and sitting did not result in significantly different airflow measurements in participants classified to be overweight, a result which suggests that an upright torso is key to effective ventilation [10]. In contrast, Palermo et al. (2005) reported that participants without past cardiac problems had airflow values that were nearly identical in both the supine and sitting positions [11]. However, the same study also showed that Forced Vital Capacity (FVC) and Forced Expiratory Volume (FEV1) values increased by 2.3% and 1.6%, respectively, from the supine to the sitting position in subjects with cardiac function difficulties [11]. Both Talwar et al. (2002) and Meysman et al. (1998) reported lower expiratory flow rates in supine than sitting postures [12,13], similar to the effects of lateral recumbancy reported by Manning et al. (1999) [14]. However, none of these studies tested intermediate torso positions, with the exemption of Lin et al. (2006) who tested an intermediate (slumped) position without back support [15].
In general, it is understood that elevations of the torso improve pulmonary performance, such that standard caregiver techniques to facilitate patient pulmonary function include upper bed (and thus patient torso) inclination of 30-45° [1,2,16,17]. This upright angle is accepted to improve pulmonary function and may also deter gastric contents from moving into the lungs and causing aspiration pneumonia [2,16,18] (although the degree to which this opinion is evidenced-based recently has been called into question [1]). Similarly, a neutral head position is accepted as being most favorable for pulmonary flow [5,6]. However, prior studies have failed to consider how torso position and head rotation may interact to influence pulmonary function, despite the fact that patients often manipulate both positional aspects simultaneously. Here, we sought to specifically assess the degree, and form, of interaction between torso flexion and head rotation in their impact on exhalation function in healthy subjects, and to model their interaction to allow prediction of their combined effects.
2. Materials and Methods
2.1. Protection of Human Subjects
This study was approved by the Institutional Review Board of the Indiana University of Pennsylvania (log # 10-015).
2.2. Equipment and Participants
A single, portable spirometer (Spirolab II; SDI Diagnostics, Inc.; Easton, MA), was used to measure pulmonary exchange. The spirometer was factory-calibrated. To verify the quality of spirometer calibration, 26 separate tests were completed using a 3.0 L pulmonary calibration syringe. For each test, the calibration syringe was operated in a sequence designed to mimic human subject FVC testing (3 normal [shallow] breath cycles, followed by 3 deep-breath maneuvers). The spirometer proved to be very accurate, with the maximum measurement error in these tests observed to be 0.07 L. The spirometer was also very precise, as test measurements exhibited very little variability (standard deviation = 0.01 L).
Pulmonary measurements from eight different testing positions were obtained from fifty-four healthy, mixed-sex subjects aged eighteen to twenty-five years old. Subjects were recruited by verbal announcement; no financial incentives were provided for study participation. Subject health and lifestyle activity levels were assessed via questionnaire.
2.3. Testing Procedure
Subject height, weight, ethnicity, sex, and age were programmed into the spirometer in order to obtain individually-specific expected values for both FEV and FVC1, via Knudson-method calculations [19,20]. The body positions and breathing maneuvers required were both described to and demonstrated for each subject prior to testing.
Subjects were tested in positions representing four different torso elevations: 0° (supine), 45° (reclining), 90° (seated upright), and 110° (forward bend). Subjects’ legs remained parallel to the floor at all torso positions. The sequence of torso positions used during testing was varied among subjects, to minimize the potential for systematic error due to a static testing order [10]. Subjects were fitted with a disposable nose clip throughout data collection.
At each torso position, subjects held the spirometer flow sensor in their right hand, and began with their head in alignment with the body axis (no rotation) and at neutral flexion. Subjects completed three deep-breath maneuvers as instructed, and then rotated their head 60° to the right, making firm contact with a head support which both constrained and maintained head rotation. In this turned-head position, subjects then completed three additional deep-breath maneuvers. Subject torso was then adjusted to a different angle, and testing resumed with the head returned to a neutral position. Each subject completed testing of the four torso positions in one experimental session lasting 20-30 min.
2.4. Measurements Obtained
The primary measurements retrieved from the spirometer for analysis included the forced vital capacity (FVC; used here as an indicator of thoracic ventilatory freedom) and the one-second forced expiratory volume (FEV1; used here as an indicator of airway resistance) [18]. Given that our subjects were healthy, unusually-low subject values (automatically flagged by the spirometer) were discarded from analysis.
2.5. Data Analysis
Spirometer data were retrieved as percentages of individually-predicted FVC and FEV1. Multiple breaths were recorded for each subject in each testing position; each individual’s highest FVC and FEV1 values for each position tested were retained for analysis. FVC and FEV1 data were grouped by position across subjects and analyzed using univariate and multivariate ANOVA (SPSS Statistics, ver. 22, IBM Corp.). Analyses focused upon (i) the relative contributions of head and body position to pulmonary performance, both separately and in interaction, and (ii) the form and magnitude of interaction between torso position and head rotation in their impacts upon exhalation performance. Where appropriate, post-hoc pairwise tests were used to examine body and head position main effects. The nominal α value for acceptance of statistical distinction was set to 0.05.
2.6. Combinatorial Models
In order to examine the ability of torso and head positions to influence pulmonary function in a combinatorial fashion, measured (independent) effect sizes for both torso and head position were evaluated against models which assessed their combined effects, in both linear and synergistic scenarios. The combined effect size expected from their linear (purely additive) interaction was the simple sum of their independent effect sizes. The minimum effect size expected under a synergistic model was estimated as ±2 SD outside of their linear combination, in order to approximate a 95% confidence interval for the detection of synergistic effects. Via this method, negatively synergistic interactions would result in a 0% effect size for the combined effects of torso angle and head rotation (e.g., their individual effects would nullify each other; Table 2). In contrast, positively synergistic interactions between torso angle and head rotation would be expected to depress pulmonary function by 18.46% for FEV1 and 18.73% for FVC (Table 2).
3. Results
3.1. Validity of Predicted Values: Analyses of Gender and Age
The eight-position testing procedure was completed with fifty-four subjects. Because the data used here were recorded as percentages of predicted pulmonary values (e.g., as proportions), we first assessed the validity of the predicted values. While pulmonary function is well-known to differ between the sexes (reviewed in [21]) and by age [18], on average neither the FVC (F1,54 = 0.64, NS) nor the FEV1 (F1,54 = 0.68, NS) proportional measurements differed between male and female participants. This suggests that the calculation of predicted pulmonary values was not biased by gender (e.g., both sexes had predicted values of 100% for their sex, and actual values close to that). Likewise, neither mean FVC (Pearson r = 0.0055, NS) nor mean FEV1 (r = -0.11, NS) proportional measurements were correlated with age, providing further confirmation of the accuracy of the Knudson method of pulmonary prediction [19,20]. Subject average FVC and FEV1 values exceeded 93% of predicted values, suggesting that participants put forth consistently high levels of respiratory effort.
3.2. Independent Effects of Torso and Head Positions
As expected [5,9], subject exhalation performance was impacted by both torso elevation and head rotation from the midline. Head rotation caused decrements in both subject FVC and FEV1, while torso angle exhibited a small influence on subject FEV1 alone (Table 1). No significant interaction between torso angle and head rotation was revealed for either measurement, suggesting that the effects of head rotation were uniform across all torso angles examined.
Table 1. Multivariate analysesa for the effects of torso angle and head rotation on exhalation performance in healthy subjects reveal that head rotation causes decrements in both subject FVC and FEV1, while torso angle exhibited a small influence on subject FEV1 alone. No significant interactions between torso angle and head rotation were revealed, suggesting that the effects of head rotation were uniform across all torso angles examined.

aGeneral linear model (GLM), IBM® SPSS® Statistics, Ver. 22
bType III Sums-of-Squares
Table 2. The measured, combined effect size of torso flexion and head rotation matches most closely those effects predicted by linear, rather than synergistic, models of their combined effects.

aMeasured difference between the max and min torso position values, for both of the head positions tested
bMeasured difference between the neutral and rotated head positions, across the four torso positions tested
cMeasured difference between the max and min overall position mean values, across the eight combinations of torso and head positions tested
dCalculated as the sum of the individual torso position and head rotation measured effect sizes, minus (2*SD) of their mean values
eCalculated as the sum of the individual torso position and head rotation measured effect sizes
fCalculated as the sum of the individual torso position and head rotation measured effect sizes, plus (2*SD) of their mean values
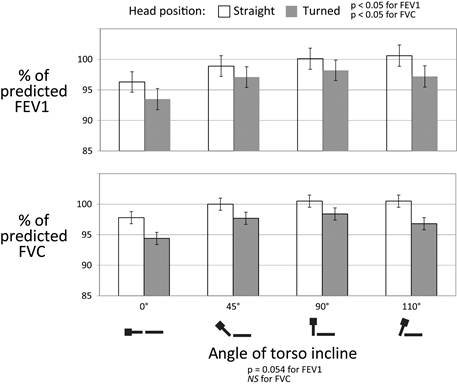
Figure 1. Body and head positioning impacts upon FEV1 (upper panel) and FVC (lower panel). Depicted here are the mean ± SEM of each individual’s highest single FVC and FEV1 measurement, averaged across all individuals tested (n = 54), for each of the 8 testing positions.
The highest average FVC values (100.5%) were obtained at both the 90° and 110° torso positions with subjects facing forward. Conversely, the lowest average FVC value (94.4%) was generated by supine subjects (0° angle) with their head turned. Subject average FVC was greater when facing forward than with the head rotated at each of the four torso angles (Figure 1). On average, FVC varied 2.9% across head rotation positions and 3.4% across torso positions (Table 2).
On average, the highest FEV1 values (100.6%) were recorded from the 110° torso position with the head aligned, while the lowest average value (93.6%) was recorded from subjects whose heads were rotated while in the supine position. As seen with FVC, rotation of the head yielded lower average FEV1 measurements than when facing forward at each of the four torso positions (Figure 1). Keeping the head in alignment yielded FEV1 values which were, on average, 2.5% greater than those measured with the head turned. On average, FEV1 values varied 4.5% due to torso position alone (Table 2).
3.3. Combined Effects of Torso and Head Positions
As described above, the head*torso ANOVA interaction term was insignificant for its effects on both FEV1 and FVC (Table 1), suggesting that torso angle and head rotation independently influence exhalation. This ANOVA interaction term reveals that head rotation and torso position effects are mutually consistent, but does not fully explain how they exist in combination. To further explore their combined effects, the measured effect sizes for torso angle and head rotation were evaluated against several models (negatively synergistic, additive (linear), and positively synergistic) of their combined effects (Table 2). Their measured combined effect is very closely approximated by the linear combination of their independent effects, differing only by 0.16% (FEV1) and 0.11% (FVC), respectively (Table 2). These analyses confirm that torso angle and head rotation are strongly independent and mutually consistent in their individual effects upon exhalation performance, and together have a linear, cumulative influence.
4. Discussion
Our data, collected from a large and relatively uniform population of healthy, young adults, contribute new information to the body of literature concerning the ability of body and head positions relevant to human patient care to influence pulmonary performance. In accord with prior studies, we have demonstrated, and measured, the independent contributions of torso position and head rotation to exhalation performance. In addition, for the first time, we have measured and modeled their combined effects, demonstrating a cumulative effect that is linear (additive), but not synergistic.
4.1. Body and Head Position Influence Pulmonary Function
Across the eight different positions tested, subject FVC and FEV1 measurements varied less than 10% from the highest to the lowest average values. Nonetheless, clear positional effects on pulmonary performance were apparent. As expected, rotation of the head away from midline depressed both subject FEV1 as well as subject FVC by small, but statistically-significant, amounts (Table 1). In contrast, changes in torso elevation had a minor effect on subject FEV1 alone. In general, the 110° and 90° positions permitted relatively more free exhalation, while the supine position consistently produced the lowest values (Figure 1).
4.2. Integration with Prior Studies
The data presented here confirm the findings of earlier studies, and provide a more-stringent assessment of torso position than is commonly assessed. Carlo et al. (1989), Behrakis et al. (1983), and Attinger et al. (1989) all demonstrated that pulmonary flow resistance tends to be higher when subjects are in a supine position than when sitting [5,8,9]; the current experiment demonstrates that this inclination effect is monotonic, as exhalation performance at an upper-torso angle of 45° was intermediate to angles less than and greater than 45°, respectively. Our results are similar to those of Lin et al. (2006), who found that FVC and FEV measurements were superior in a fully seated position over those of an intermediate, "slumped" position [15]. For most measurements, this inclination effect culminated at 90°, with little or no advantage gained by further torso flexion to 110°. That consistently lower pulmonary values were obtained from subjects in the head-turned positions agrees with the report by Carlo et al. (1989), which reported high airflow resistance in subjects whose head was flexed away from the neutral position [5].
4.3. Subject Characteristics
While numerous subjects (19/54) self-reported minor pulmonary deficits at some point in their lives (including asthma [n = 13] and allergies [n = 7]), the pulmonary problems reported in this young population tended to be mild and/or acute, rather than significant or chronic. 16 subjects reported some history of inhaler usage to improve pulmonary function. A total of 22 subjects reported some history of smoking, with only 8 subjects self-reporting having sustained, substantial (>1 pack / wk) cigarette use at any point in their lives.
As a group, subjects reporting pulmonary ailments (F = 1.21), inhaler use (F = 0.98), or any history of smoking (F = 1.70) were indistinguishable from the rest of the subject pool (all NS). The pulmonary performance of those subjects (n = 8) with a significant smoking history (sustained use of > 1 pack of cigarettes / wk) was reduced relative to the rest of the subject pool (F = 2.00, p < 0.05), with lower average FEV1 and FVC in each of the eight positions tested here. However, these subjects nonetheless exhibited the same positional effects seen in the rest of the subject pool, with all pulmonary measurements reduced in head-turned positions relative to head-aligned positions. As did subjects with little or no smoking history, subjects with a history of heavy smoking exhibited reduced pulmonary performance in the supine position and exhibited their greatest performance at either the 45° (FVC) or 90° (FEV1) torso elevations. No single subject or sub-group exhibited results which broadly differed from the group as a whole, suggesting that the pattern of results reported here is robust to slight differences in subject pulmonary health.
4.4. Application to Patient Care
Drakulovic et al. (1999) found that a simple elevation of head of bed to 45o resulted in a dramatic reduction in VAP (ventilation associated pneumonia) incidence and a trend toward reduced mortality [22]. Nonetheless, a recent survey by the University Hospital Consortium revealed that compliance with the simple, no-cost intervention of elevating the head is woefully low [23]. Further, it is commonly accepted that patient head of bed (HOB) is typically elevated to a lesser degree. For example, Heyland et al. (2002) reported an average HOB elevation of 29o (in critically-ill, bowel-fed subjects) [24], similar to the HOB values reported by Llaurado-Serraa et al. (2015) for mechanically-ventilated patients [25]. These HOB values are perhaps routine, but are less than the 30° minimum HOB angle recommended by a recent caregiver consortium for mechanically-ventilated patients [1]. This lack of compliance represents, in part, the need to balance aspiration risk with other care considerations, such as pressure ulcer risk in long-term bedded subjects (recently reviewed in Metheny and Frantz [2013]) [26]. Nonetheless our data demonstrate that strict attention to torso and head position can be advantageous for patients whose pulmonary clearance is priority. Caregiver attention must focus specifically upon patient position itself, however, as patient migration away from an elevated HOB position causes a reduction in patient torso angle from that of the HOB angle [17].
4.5. Our Data Refine the Most Beneficial Torso and Head Positions with Respect to Pulmonary Function
With less than a 2% difference in average FVC and FEV1 values in the forward-facing forward position between the 45° and the 90° body postures, there is little evidence in our data to suggest that, for most patients, a fully-upright (while seated) posture would provide substantial improvements in pulmonary function over an upper body elevation to 45° above the horizontal. However, that pulmonary performance gains tended to be incremental between the 0° and 90° positions suggests that higher levels of elevation may be warranted in those patients for whom ventilatory effectiveness is paramount, and further suggests that even slight HOB elevation may provide some benefit.
The reduction in pulmonary function caused by rotation of a subject’s head away from the midline, seen in both measurements and across all torso positions tested, argues for consideration of patient head rotation for patients in primary care settings whose pulmonary function is impaired. Rotation of the head while inclined in a bed is perhaps a natural response during relaxation or sleep, but also is one that might easily be checked with supportive cushioning and caregiver attention. While Hilinski and Stark (2006) noted the value of maintaining the torso angle of patients [16], this study has demonstrated the additional and related benefit in keeping a patient’s head in a forward-facing position.
It is possible that patient risk of nosocomial pneumonia during non-assisted ventilation and during NIV (noninvasive mechanical ventilation) may also be reduced with the utilization of a device that can maintain a patient’s head position to assist in maximizing airflow. With more patients contracting pneumonia than any other infection while in hospital intensive care units [2], the implementation of such a device could be advantageous to a useful number of patients. Our data support the premise of Dean (1985), who suggested that significant attention should be given to individualized patient posturing in order to maximize pulmonary effectiveness [27], especially when strict attention (including electronic, real-time monitoring) improves compliance with accepted recommendations for patient positioning [25,28].
5. Conclusions
We have demonstrated the ability of both torso flexion and head rotation to impair patient exhalation function, and also demonstrate that their combined effects are additive, but not synergistic. While the magnitudes of the individual and combined impacts on pulmonary exchange are modest, their effects are statistically significant and robust across multiple positions and subjects. This suggests that the pulmonary benefits of attention to both torso flexion and head rotation may easily be gained by most subjects, and may prove very advantageous in those subjects whose pulmonary function is most critical.
Acknowledgements
This research was supported by research awards from the Indiana University of Pennsylvania Faculty Senate (to PMN) and School of Graduate Studies and Research (to ZJA), used to purchase equipment and supplies for this study. The authors collectively report no conflicts of interest in the conduct or publication of this research.
References
- Niël-Weise, B.S., et al., An evidence-based recommendation on bed head elevation for mechanically ventilated patients. Critical Care, 2011. 15(2): p. R111-R111.
- Balonov, K., et al., A novel method of continuous measurement of head of bed elevation in ventilated patients. Intensive care medicine, 2007. 33(6): p. 1050-4.
- Newhouse, M.T. and J. Bienenstock, Respiratory tract defense mechanisms, in Textbook of Pulmonary Disease, G.L. Baum and E. Wolinsky, Editors. 1989, Little, Brown and Co. p. 21–47.
- Mulvihill, M.L., et al., Human diseases: a systematic approach. 5th ed. 2001, Upper Saddle River, NJ: Prentice Hall.
- Carlo, W.A., et al., Neck and body position effects on pulmonary mechanics in infants. Pediatrics, 1989. 84(4): p. 670-4.
- Badr, C., M.R. Elkins, and E.R. Ellis, the effect of body position on maximal expiratory pressure and flow. The Australian journal of physiotherapy, 2002. 48(2): p. 95-102.
- Melissinos, C.G. and J. Mead, Maximum expiratory flow changes induced by longitudinal tension on trachea in normal subjects. Journal of Applied Physiology: Respiratory, Environmental and Exercise Physiology, 1977. 43(3): p. 537-44.
- Behrakis, P.K., et al., Lung mechanics in sitting and horizontal body positions. Chest, 1983. 83(4): p. 643-6.
- Attinger, E.O., R.G. Monroe, and M.S. Segal, The mechanics of breathing in different body positions. I. In normal subjects. The Journal of clinical investigation, 1956.35(8): p. 904-11.
- Gudmundsson, G., M. Cerveny, and D.M. Shasby, Spirometric values in obese individuals. Effects of body position. American journal of respiratory and critical care medicine, 1997. 156(3 Pt 1): p. 998-9.
- Palermo, P., et al., Lateral decubitus position generates discomfort and worsens lung function in chronic heart failure. Chest, 2005. 128(3): p. 1511-6.
- Meysman, M. and W. Vincken, Effect of body posture on spirometric values and upper airway obstruction indices derived from the flow-volume loop in young nonobese subjects. Chest, 1998. 114(4): p. 1042-7.
- Talwar, A., S. Sood, and J. Sethi, Effect of body posture on dynamic lung functions in young non-obese Indian subjects. Indian journal of medical sciences, 2002. 56(12): p. 607-12.
- Manning, F., et al., Effects of side lying on lung function in older individuals. Physical therapy, 1999. 79(5): p. 456-66.
- Lin, F., et al., Effect of different sitting postures on lung capacity, expiratory flow, and lumbar lordosis. Archives of physical medicine and rehabilitation, 2006. 87(4): p. 504-9.
- Hilinski, A.M. and M.L. Stark, Memory aide to reduce the incidence of ventilator-associated pneumonia. Critical Care Nurse, 2006. 26(5): p. 2.
- Wiggermann, N., et al., The effect of patient migration in bed on torso elevation. Nurs Res, 2015. 64(3): p. 221-5.
- Des Jardins, T., et al., Clinical manifestations and assessment of respiratory disease. 4th ed. 2002, St. Louis, MO: Mosby.
- NIOSH Spirometry Training Guide. 2003, Atlanta, GA: Universities Occupational Safety and Health Education Resource Center.
- SDI Diagnostics, I., Spirolab II Users' Manual. 2001, Easton, MA.
- Harms, C.A., Does gender affect pulmonary function and exercise capacity? Respiratory Physiology & Neurobiology, 2006. 151(2–3): p. 124-131.
- Drakulovic, M.B., et al., Supine body position as a risk factor for nosocomial pneumonia in mechanically ventilated patients: a randomised trial. Lancet, 1999. 354(9193): p. 1851-8.
- Koenig, S.M. and J.D. Truwit, Ventilator-associated pneumonia: Diagnosis, treatment, and prevention. Clinical Microbiology Reviews, 2006. 19(4): p. 637-+.
- Heyland, D.K., et al., Optimizing the benefits and minimizing the risks of enteral nutrition in the critically ill: role of small bowel feeding. JPEN J Parenter Enteral Nutr, 2002. 26(6 Suppl): p. S51-5; discussion S56-7.
- Llaurado-Serraa, M., et al., Evaluation of head-of-bed elevation compliance in critically ill patients under mechanical ventilation in a polyvalent intensive care unit. Medicina Intensiva, 2015. 39: p. 329-36.
- Metheny, N.A. and R.A. Frantz, Head-of-Bed Elevation in Critically Ill Patients: A Review. Critical Care Nurse, 2013. 33(3): p. 53-65.
- Dean, E., Effect of body position on pulmonary function. Physical therapy, 1985. 65(5): p. 613-8.
- Wolken, R.F., et al., Observational Study of Head of Bed Elevation Adherence Using a Continuous Monitoring System in a Medical Intensive Care Unit. Respiratory Care, 2012. 57(4): p. 537-543.