Qualitative By-Products Analysis of Non-thermal Plasma Decomposition of Toluene and Xylene from Wastewater Using Fourier Transform Infrared Spectroscopy
Abdullahi Mohammed Evuti
Department of Chemical Engineering, University of Abuja, Abuja, Nigeria
Email address
Citation
Abdullahi Mohammed Evuti. Qualitative By-Products Analysis of Non-thermal Plasma Decomposition of Toluene and Xylene from Wastewater Using Fourier Transform Infrared Spectroscopy. American Journal of Environmental Engineering and Science. Vol. 3, No. 4, 2016, pp. 90-99.
Abstract
Information on the by-product distribution in plasma decomposition of volatile organic compounds is an important prerequisite to the understanding of the chemistry of plasma decomposition process and to achieve complete oxidation at a minimum energy cost. This research focused on analysis of by-products of the decomposition of toluene and xylene stripped from wastewater using ferroelectric packed bed non thermal plasma reactor operated at 15.68, 14 and 12.32 kV and fixed discharge gap and flow rate of 25 mm and 2.36 L/min respectively. The by-products were qualitatively analyzed with Fourier transform infrared spectroscopy. CO, CO2, H2O and N2O were identified as the by- products. Also, CO formation decreased with increase in applied voltage.
Keywords
Wastewater, Volatile Organic Compounds, Air Stripper, Non-thermal Plasma Reactor, Fourier Transform Infrared Spectroscopy
1. Introduction
Effluents from industries, contaminated ground-water and hazardous landfill leachate release volatile organic compounds (VOCs) into the surrounding. A study of VOCs in drinking water in peninsular Malaysia detected 54 different VOCs species in the samples analysed from 11 states which were attributed to improper disposal practice [1]. The negative effects of volatile organic compounds (VOCs) on humans and the environment remains a global challenge [2-3]. These include stratospheric ozone depletion, ground level photochemical ozone formation, toxic or carcinogenic human health effects, enhancing the global greenhouse effect and accumulation and persistence in the environment [4-9]. Therefore abatement of VOCs has to be handled with highly efficient and low cost process [8-9].
Non-thermal plasma generated in electrical discharges is attractive for VOC removal from contaminated air streams, since it can be operated at room temperature and atmospheric pressure, over a wide range of gas flow rates and concentrations [10-13]. During the process, electrons dissociate molecular oxygen to atomic oxygen which reacts with VOCs. More also, oxidative species such as O3 and OH- produced by the initial oxidation have an effect on further reaction of VOCs [14]. The desirable decomposition of VOCs is to form CO2, and H2O, which are thermochemically stable in this reaction system; however, the non-equilibrium state in the plasma produces CO and high molecular species such as methane (CH4), acetylene (C2H2), ethylene (C2H4) have been observed from some studies [15-16]. The ratio of CO to CO2 was found to be in the range of 0.6–1.0 when this system was operated under standard conditions. This however increases with increase in electric energy. Other byproducts of plasma decomposition are nitrogen oxides (NOx), N2O, O3, Cyanide (CHN) and aerosols [9, 17].
Identification and quantification of all the by-products of NTP treatment is an important prerequisite to the understanding of the chemistry of plasma decomposition process and to achieve complete oxidation at a minimum energy cost [9, 18]. This information can consequently help to improve the design of NTP systems. More also, the knowledge of the byproducts and plasma mechanism helps in the choice of appropriate catalysts to enhance plasma decomposition processes [9-10]. In recent times, the application of ferroelectric packed bed non thermal plasma reactor for VOCs abatement have been studied but there is need for further attention to the determination of the possible by-products formed due to incomplete oxidation particularly for VOCs from wastewater [19-24]. Therefore, in this paper, Fourier transform infrared (FT-IR) spectroscopy has been applied to quantitatively and qualitatively analyze the by-products of toluene abatement with a ferroelectric packed bed non thermal plasma reactor. The effects of discharge gap, applied voltage and flow rate on the toluene decomposition efficiency were also investigated.
2. Materials and Methods
2.1. Materials
Toluene and xylene were obtained from Merck Sdn Bhd. Malaysia with greater than 99.5% purity. Synthetic wastewater containing 1500 ppm of toluene and xylenes was prepared by mixing 1.73ml, and 1.75ml of toluene and xylene into 1 litre of distilled water respectively. The experimental set up is shown in Figure 1. It consist of a custom-made pilot scale packed column air stripper (Model 2T4H) from Branch Environmental Corporation USA made of a 1.5 m stainless steel tube of 0.05 m internal diameter filled with 6 mm ceramic raschig rings packing. The height of the packing is 1.15 m which is equivalent to a packing volume of 2.26 x 10-3 m3. The ferroelectric packed bed NTP reactor consists of a pyrex glass tube of 1 inch internal diameter and standard length and 3mm diameter barium titanate (BaTiO3) pellets (dielectric constant of 10,000) from Fuji Titanium Industry Co., Ltd, Japan (Figure 2) as dielectric material packed in between the two electrodes made from stainless steel. The electrodes and BaTiO3 pellets were supported with two funnel head stainless steel tubes. O-rings smeared with vacuum grease were used to fix the packed-bed in between the two mesh electrodes and to ensure that it is properly sealed. Teflon caps equipped with O-rings were then used to seal both ends of the Pyrex tube to create a properly confined area in the Pyrex tube. High voltage electric connectors were fixed to the two ends of the stainless steel tube.
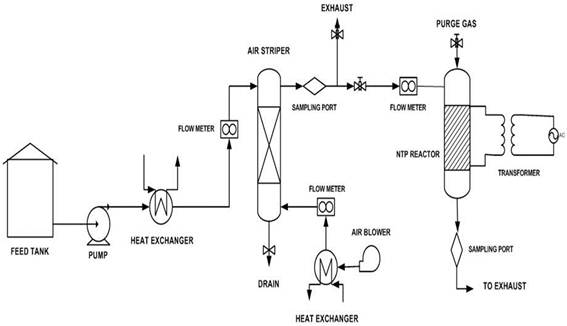
Figure 1. Process flow diagram of the integrated air stripping-NTP reactor system.
The power supply system for NTP reactor is shown in Figure 2. A transformer, with input power of 240 V and 50 Hz frequency was used to step up the input voltage of 20 kV, 20 kHz (Plasma Clean) power supply. A voltage probe with 1:1000 voltage ratios was used to measure the high voltage gained by the transformer. Voltage and current was recorded using a PC based Pico scope.
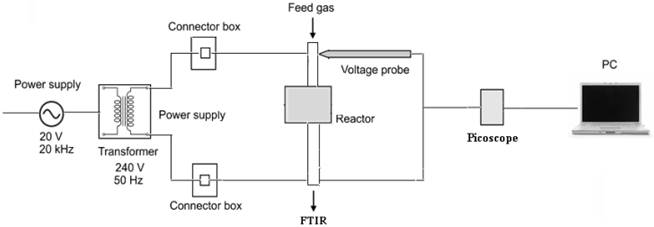
Figure 2. Power system operation setup of the packed-bed NTP reactor.
2.2. Methods
To remove the toluene and xylene from the wastewater using the air stripper, the air flow rate (7.08 L/min) was set using a rotameter and the wastewater inlet was also set to 0.12 L/min by adjusting the rotameter while the wastewater and air heaters were set to 50°C. The air and contaminated water were then pumped into the air stripper in counter-current operation. The treated wastewater was collected at the bottom while VOC rich air which comes out at the top of the column was sent to the non-thermal plasma reactor. The decomposition of toluene and xylene in ferroelectric packed bed NTP was conducted at applied voltages of 12.32, 14 and 15.68 kV and fixed discharge gap and flow rate of 25 mm and 2.36 L/min respectively. A high voltage probe was used to measure the applied voltage and the current was measured using digital Pico scope.
The concentrations of toluene and xylene in the outlet gas from air stripper were first determined with a frontier FT-IR spectrometer (Perkin Elmer) coupled with cyclone gas cell accessory (cycloneTM C5) manufactured by Specac Ltd. The gas streams were passed into multiple pass optical gas cell (Specac Ltd) with a white-type mirror arrangement with an optical path length set at 8m. Sample spectrum for each operating condition was captured at room temperature and atmospheric pressure at a spectra resolution of 1cm-1. This was then followed by the determination of decomposition efficiency of toluene and xylene and by-products from non-thermal plasma treatment by passing gas streams from non-thermal plasma through the FTIR as explained earlier. Concentrations of toluene and xylene in each spectrum were determined by integrating the area under the peaks using Perkin Elmer spectrum standard v10.4.0 software and then comparing with standard spectra produced by Pacific Northwest National Laboratories.
The gas concentration from the integrated area of the gas absorption band was then calculated by using Equation (1).
(1)
where Nstd, Lstd (given as 1 meter), Lmeas in Equation (1) represent the concentration of the standard gas, optical path length of the standard gas measurement, and optical path length of the measured spectrum, respectively. The Nstd for toluene and xylene were given as 100ppm
The destruction efficiencies of toluene and xylene (
were calculated as follows:
(2)
3. Results and Discussion
3.1. Decomposition of Toluene and Xylene in Packed Bed NTP Reactor
The main goal of the integrated system is not only to decrease the pollution from wastewater, but to also avoid shifting water pollution to air pollution by returning clean air to the atmosphere. The treated wastewater from the air stripping operation was collected at the bottom while VOC rich air which comes out at the top of the column was sent to the non-thermal plasma reactor. The concentrations of toluene and xylene in the exit air stream from air stripper are shown in Table 1. This shows a high removal efficiency of both toluene and xylene from the air stripper.
Table 1. Toluene and xylene gas concentrations after air stripping extracted from the FTIR spectra.
Species | Integration range (cm-1) | INTexp | INTstd | Concentration (ppm) |
Toluene | 3145.4 – 2824.3 | 258.63 | 2.16 | 1496.70 |
Xylene | 3162.9 - 2811.0 | 237.94 | 2.24 | 1327.79 |
Therefore, the inlet concentrations of toluene and xylene into the packed bed NTP reactor were 1496.70 ppm and 1327.79 ppm respectively. One interesting observation using the developed integrated air stripping and packed bed NTP reactor system was the possibility of plasma generation using VOCs rich air stream directly from air stripper without any need for another carrier gas such as argon or nitrogen. This shows the compatibility of the integrated system.
Table 2. Percentage removal efficiencies after decomposition of toluene and xylene in packed bed NTP reactor.
Applied voltage (kV) | Concentration after plasma treatment (ppm) | Removal efficiency (%) |
Toluene | Xylene | Toluene | Xylene |
12.32 | 601.45 | 568.25 | 59.81 | 57.2 |
13.00 | 520.54 | 485.10 | 65.2 | 63.5 |
14.00 | 480.84 | 447.27 | 67.87 | 66.3 |
15.00 | 281.94 | 289.30 | 81.2 | 78.2 |
15.68 | 20.58 | 174.05 | 92.00 | 86.89 |
The results in Table 2 show that the percentage decomposition increased from 59.8 to 92% and 57.2 to 86.9% as the voltage was increased from 12.32 to 15.68 kV for toluene and xylene respectively. This can be attributed to increase in electron density as discharge current and voltage increases. In addition, the electric field and mean electron energy gets higher as the applied voltage is raised [13, 19].
3.2. Infrared Spectra of Toluene and Xylene
The characteristics infrared band for aromatic compounds is shown in Table 3.
Table 3. Characteristics infrared band for aromatic compounds [25].
Wavenumbers range (cm-1) | Vibration |
3100-3000 | C-H stretch |
2000-1665 | Overtones (weak bands) |
1600-1585 | C-C stretch (in ring) |
1500-1400 | C-C stretch (in ring) |
1088.4-1031 | C-H stretch (in plane) |
900-675 | C-H stretch (out of plane) |
Figures 3 and 4 show zoomed spectra of air stream from air stripper operated at air-water ratio of 60 and column temperature of 50°C containing toluene and xylene before treatment in the NTP reactor respectively. Figure 3 shows three compounds which include a broad toluene absorption band (3152-2829 cm-1) with identified center of 2975 cm-1, a broad water absorption band from wave length of 4000 – 3500 cm-1 and 2000 – 1271.6 cm-1 signifying that toluene rich air from air stripper contains significant amount of water vapour and CO2 absorption band from 2396.2-2283.3 cm-1. Similarly, Figure 4 shows the presence of water and carbon dioxide along with a broad xylene absorption band (3162.9-2811 cm-1) with identified center of 2972 cm-1.
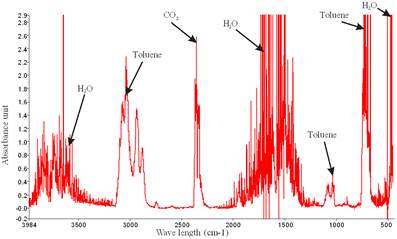
Figure 3. Spectrum of air stream from air stripper operated at air-water ratio of 60 and column temperature of 50°C containing toluene.
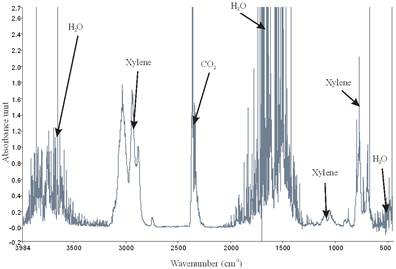
Figure 4. Spectrum of air stream from air stripper operated at air-water ratio of 60 and column temperature of 50°C containing xylene.
3.3. Overview and Discussion of By-Product Identification
a) Byproducts of toluene decomposition
From the zoomed recorded spectrum of the decomposition of toluene in ferroelectric packed NTP reactor shown in Figure 5, four gaseous byproducts (CO, CO2, H2O and N2O) were detected. This include the presence of residual toluene absorption band (3152 - 2829 cm-1) with identified center of 2975 cm-1 since 100% decomposition was not achieved and a broad water absorption band from wave length of 4000 – 3500 cm-1 and 2000 – 1271.6 cm-1. Others are CO2 absorption band from 2396.2-2283.3 cm-1, CO absorption band from 2050-2240 cm-1 with identified center of 2143 cm-1 and N2O absorption band from 2170-2260 cm-1with identified center of 2226 cm-1 as shown in a zoomed spectrum in Figure 6. Similar results on toluene decomposition have been reported [26]. According to Liang et al., toluene because of its simple configuration of molecule produces few by-products after NTP treatment [27]. This has been represented in a simple mechanism by Chang and Lin [28] as follows.
Initiation:
(3)
Decomposition:
(4)
Completing reaction:
(5)
(6)
(7)
where p is the actual input power, R the active species such as electrons and hydrocarbon radicals and ions, Y the intermediate hydrocarbon species and M the third body (N2, O2). However, Zhu et al. reported the formation other byproducts such as ozone, aldehyde, amide, alcohols and benzene derivative during decomposition of toluene in a dielectric barrier discharge reactor in combination with catalyst [24].
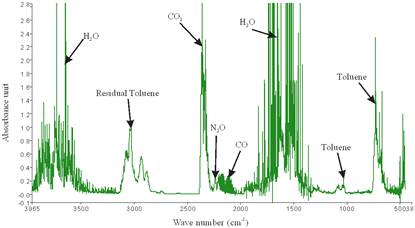
Figure 5. Spectrum of decomposition of toluene in NTP reactor showing the by-products at applied voltages of 12.32 kV, discharge gap of 25 mm and flow rate of 3.54 L/min.
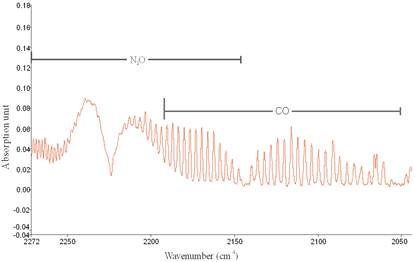
Figure 6. Zoomed Spectrum showing N2O and CO formation from toluene decomposition at applied voltages of 12.32 kV, discharge gap of 25 mm and flow rate of 3.54 L/min.
A large number of high energy electrons, ions and free radicals are produced in the NTP reaction system and multiple chemical processes such as excitation, ionization and dissociation are initiated by collisions between background molecules (N2, O2, H2O) and these highly energetic electrons. This leads to the formation of active, unstable species (radicals, ions, photons) which are responsible for the decomposition of VOCs [9, 14, 22, 24]. Firstly, the high energy electrons react with oxygen in air as follows [24]:
(8)
(9)
The oxygen free radical groups react with oxygen and other molecules to form ozone:
(10)
At the same time, the high energy electrons react with H2O and N2 in gaseous phase:
(11)
(12)
(13)
(14)
Where
,
,
represents unstable species.
Toluene bond energy between the carbon of benzene ring and the carbon of the substituent radical is 3.6 eV, which is lower than that of carbon-carbon bond (5.0-5.3 eV) or hydrocarbon bond (4.9 eV). As a hydrogen atom in a benzene ring is replaced by a methyl radical to form toluene, the newly formed bond is less stable and the most vulnerable [21]. Several possible reactions equations of the process of toluene decomposition in NTP reactor have been proposed [24, 26, 27]. The reaction begins with the rupture of benzene ring requiring energy of 5.5 eV for c=c and 3.6 eV for c-c bond and then followed by a series of oxidation reaction [27]. For the case of toluene decomposition in humid air in NTP reactor, Kohno et al. reported that the reaction of toluene with O, OH and O3 are insignificant because of their very low reaction rates constants as shown by Equations 14-16 [26].
(15)
(16)
(17)
However, charge reactions of toluene with ions and recombination of toluene ions and electrons are significant as shown by Equations 17-18.
(18)
(19)
The dissociated by-products are also reacted with oxygen, nitrogen and hydrogen free radicals produced by plasma processes where O3, CO, CO2, NOx, H2O are also observed as discharge byproduct. Hence, the mechanism of toluene destruction by an energetic electron induced plasma process can be summarized as follows [24, 26]:
i. Dissociation and ionization of O2, N2 and toluene by electrons to form O, O3, N, H, hydrocarbon radicals and ions;
ii. Formation of OH radicals from radical atom-molecule and ion-molecule reactions;
iii. Dissociation of toluene by OH radicals and negative ions to form hydrocarbons;
iv. Oxidation of hydrocarbons to form CO, CO2 and H2O;
v. Oxidation of nitrogen and oxygen to form NOX, and O3, and finally trace N2O and HNO3 formations;
vi. Formation of aerosol particles by ion induced aerosol formations from hydrocarbons and nitrates.
It is important to note that although it was possible to identify the main by-products, there might be others which cannot be detected because bands of water cover big part of the spectrum and, therefore, could mask other substances as well. Similar observations have also been reported in some previous works [29-30].
b) Byproducts of xylene decomposition
Figure 7 is the FTIR spectrum showing the by-product of the decomposition of xylene in NTP reactor. Similar to toluene, the presence of broad water absorption band from wave length of 4000 – 3500 cm-1 and 2000 – 1271.6 cm-1 was also observed in the detection of xylene decomposition byproducts. The figure also shows the presence of residual xylene absorption band (3162.9-2811 cm-1) with identified center of 2972 cm-1 since 100% decomposition was not achieved. Others include CO2 absorption band from 2396.2-2283.3 cm-1 and CO absorption band with identified center of 2143 cm-1 and N2O absorption band with identified center of 2226 cm-1. This result is in agreement with that obtained by Kuroki et al. [30].
In another studies using electron beam induced NTP reactor, Hakoda et al. also detected formic acid (HCOOH) along with CO, CO2 and O3. when xylene was decomposed [3]. More also Piroi et al. (2008) detected only CO and CO2 as the major gaseous product when xylene was decomposed using dielectric barrier discharge reactor. Similarly, Ogata et al. reported that the decomposition of xylene in packed bed NTP reactor produced over 98% CO and CO2 with only small amount of CH4 and C2H2 by-products [21]. Ogata et al. developed a model for the decomposition mechanism of aromatics in NTP reactor. The model shows that important reactions at the initial stage of the diluted hydrocarbon decomposition follow one or more of the following steps [21].
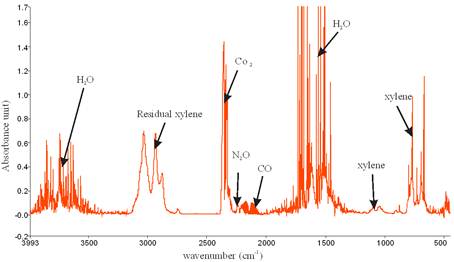
Figure 7. Spectrum of decomposition of xylene in NTP reactor showing the by-products at 12.32 kV, discharge gap of 25 mm and flow rate of 3.54 L/min.
i. Low-energy electron dissociative attachment
(20)
ii. Dissociative recombination
(21)
(22)
(23)
iii. Chemical reaction by activated species
(24)
(25)
On the basis of the by-products (CO, CO2, H2O and N2O) observed from the decomposition of xylene, it can be proposed that the reaction mechanisms proceeds through the above listed steps beginning with the dissociation of C-CH3 bond. It is important to note that although it was possible to identify the main by-products, there might be others which cannot be detected because bands of water cover big part of the spectrum and, therefore, could mask other substances as well. Similar observations have also been reported in some previous works [29-30]
3.4. Effect of Applied Voltage on N2O and CO Formation During Decomposition of Toluene and Xylene
The results of the investigation of the effect of applied voltage on N2O and CO formations from toluene and xylene decomposition are shown in Figures 8 and 9. Carbon monoxide is produced from the partial oxidation of carbon containing compounds; it forms when there is not enough oxygen to produce carbon dioxide (CO2). It can also be formed from the oxidation of CO2 as shown in Equation (26).
(26)
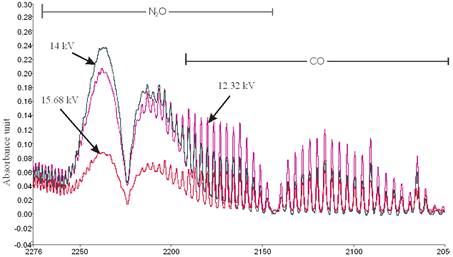
Figure 8. N2O and CO formation from toluene decomposition at different applied voltages.
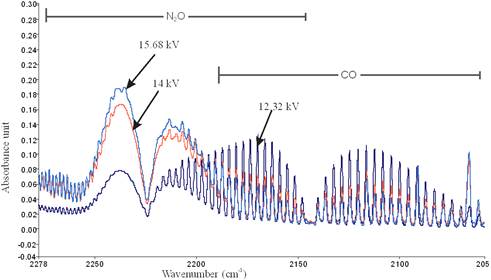
Figure 9. N2O and CO formation from xylene decomposition at different applied voltages.
The CO formation was observed to increase with decrease in applied voltage for both toluene and xylene. This is in good agreement with previous studies [32-33]. Holzer et al. reported that the selectively to CO2 formation during the decomposition of toluene in a ferroelectric packed bed reactor increases with applied voltage thus less CO are formed at high voltage [32]. Also, Yu-fang et al. explained that this phenomenon is due to that the electron energy is not high enough for the oxygen to be ionized in the reaction when electric field strength is low [33]. Therefore only a part of toluene is oxidized to CO whereas when the applied voltage is high CO is oxidized to CO2. More also, Ogata, et al. reported that CO2 formation increases with increase in electric energy [16]. However, in another studies using dielectric barrier discharge reactor Chang and Lin also reported that the selectivity of CO also increases with applied voltage during toluene decomposition [28].
In NTP operation using air as carrier gas, excited nitrogen species in air react with oxygen to form nitrogen oxides, like NO, NO2, NO3, N2O5 N2O. Nitrous oxide N2O is the initial oxide formed within 100 ns after the initiation of microdischarge. The main reaction leading to N2O formation is involving metastable excited N2 molecule [29]:
(27)
The formation of nitrogen oxides in NTP process has the following disadvantages [29-30]:
i. Nitrogen oxides are undesirable in atmosphere because they form smog, participate in ozone depletion, and N2O is a greenhouse gas with global warming potential 298.
ii. Nitrogen oxide formation consumes a lot of energy, decreasing plasma efficiency, but in air it cannot be avoided
Another reaction path for the formation of N2O begins with the reaction of atomic oxygen with nitrogen as given in Equations 27-30.
(28)
(29)
(30)
(31)
The CO formation was observed to increase with decrease in applied voltage for both toluene and xylene at fixed discharge gap and flow rate. This can be further understood from the concentrations of N2O and CO formed from the decomposition of toluene and xylene in packed NTP reactor at fixed discharge gap of 25mm and flow rate of 3.54 L/min shown in Table 4. The concentrations of N2O and CO were determined by performing integration over the non-overlapping areas of the absorption bands. The selected integration ranges were 2144.2 – 2050 cm-1 for CO and 2260 – 2224 cm-1 for N2O.
Table 4. Concentrations of N2O and CO formed from toluene and xylene decomposition in packed bed NTP reactor at discharge gap of 25mm and flow rate of 3.54 L/min.
Applied Voltage (kV) | Toluene | Xylene |
CO (ppm) | N2O (ppm) | CO (ppm) | N2O (ppm) |
12.32 | 66.4 | 11.5 | 52.7 | 4.2 |
14 | 42.3 | 14.3 | 40.1 | 8.9 |
15.68 | 32.7 | 4.6 | 36.6 | 10.4 |
The concentration of CO formed decreased from 66.4 to 32 ppm as applied voltage was increased from 12.32 to 15.68 kV for toluene while a decrease from 52.7 to 36.6 ppm was observed for xylene under the same condition. This is in good agreement with previous studies (Holzer et al., and Yu-fang et al., [32, 33]. Holzer et al. [32] reported that the selectively to CO2 formation during the decomposition of toluene in a ferroelectric packed bed reactor increases with applied voltage thus less CO are formed at high voltage. Also, Yu-fang et al. [33] explained that this phenomenon is due to that the electron energy is not high enough for the oxygen to be ionized in the reaction when electric field strength is low. Therefore only a part of toluene is oxidized to CO whereas when the applied voltage is high CO is oxidized to CO2. More also, Ogata et al. [16] reported that CO2 formation increases with increase in electric energy. However, in another studies using dielectric barrier discharge reactor Chang and Lin [28], also reported that the selectivity of CO also increases with applied voltage during toluene decomposition.
From Figures 6.9 and 6.10, N2O formation was observed to increase as the applied voltage was increased for both toluene and xylene. This can also be seen from the concentrations of N2O and CO formed from the decomposition of toluene and xylene in packed NTP reactor at fixed discharge gap of 25mm and flow rate of 3.54 L/min shown in Table 4. The concentrations of N2O formed increased from 4.2 to 10.4 ppm as applied voltage was increased from 12.32 to 15.68 kV for xylene. While an increase from 11.5 ppm to 14.3 ppm was observed for toluene as the applied voltage was increased from 12.38 kV to 14 kV. However, a low value of formation of N2O (4.6 ppm) was obtained for at 15.68 kV. Similar results were reported by Li et al. [34] and Kim et al. [35]. Li et al. observed that formation of N2O increased with applied voltage during the decomposition of benzene, toluene and xylene using streamer discharge [34]. Kim et al. further reported that the formation of N2O reduced when the decomposition was carried out in the presence of catalyst [35].
N2O formation was observed to increase as the applied voltage increase for xylene. Similar results were reported by Li et al. and Kim et al. [34-35]. Li et al. observed that formation of N2O increased with applied voltage during the decomposition of benzene, toluene and xylene using streamer discharge [34]. Kim et al. further reported that the formation of N2O reduced when the decomposition was carried out in the presence of catalyst [35]. However, contrary result was obtained for toluene with lowest N2O formation at the highest applied voltage.
4. Conclusion
In this paper, the decomposition of toluene and xylene stripped from wastewater was studied with ferroelectric packed bed NTP reactor operated at 15.68, 14 and 12.32 kV and fixed discharge gap and flow rate of 25 mm and 2.36 L/min respectively. The by-products were qualitatively analyzed with FTIR. CO, CO2, H2O and N2O were identified as the oxidation products. The CO formation increases with decrease in applied voltage for both toluene and xylene while N2O formation was observed to increase as the applied voltage increase for xylene but decrease with increase in applied voltage for toluene.
Acknowledgements
The authors would like to express their sincere appreciation to Universiti Teknologi, Malaysia (UTM) for financial support under exploratory research grant scheme (ERGS) and to Professor Koichi Takaki of Iwate University Japan for providing the barium titanate used for this research.
References
- Abdullahi MP, Chian SS. Chlorinated and non chlorinated volatile organic compounds (VOCs) in drinking water of peninsular Malaysia. Sains Malaysiana 2011; 40:11 1255-1261.
- Lelieveld J, Hoor P, Jöckel P, Pozzer A, Hadjinicolaou P, Cammas JP, Beirle S. Severe ozone air pollution in the Persian Gulf region. Atmos Chem and Phys 2009; 9:4 1393-1406.
- Wuebbles DJE, Hayhoe K. Atmospheric methane and global change. Earth-Sci Rev 2002; 57:177-210.
- Derwent RG. Sources, distributions and fates of VOCs in the atmosphere. In Hester RE, Harrison RM, Derwent RG editors Volatile organic compounds in the atmospheres. The Royal Society of Chemistry UK 1995; 1-15.
- Goldstein AH, Galbally IE. Known and unexplored organic Constituents in the earth’s atmosphere. J of Enviro Sci and Technol 2007; 41:5 1515-1521.
- Inoue K, Okano H, Yamagata Y, Muraoka K, Teraoka Y. Performance Tests of newly developed adsorption/plasma combined system for decomposition of volatile organic compounds under continuous flow Conditions. J of Environ Sci 2011; 23:1 139-144.
- Zhu T, Li J, Lang Y, Ma G. Decomposition of benzene by non-thermal processing: Photocatalyst and ozone effect. Int J of Enviro Sci and Technol 2008; 5:3 375-384.
- Subrahmanyam C. Catalytic non-thermal plasma reactor for total oxidation of volatile organic compounds. Indian J of Chem 2009; 48A:1062-1068.
- Vandenbroucke AM, Dinh MTN, Giraudon J, Morent R, Geyter ND, Lamonier J, Leys C. Qualitative by-product identification of plasma-assisted TCE abatement by mass spectrometry and FT-IR. Plasma Chem Plasma Process 2011; 31:707-718.
- Magureanu M, Piroi D, Mandache NB, Parvulescu VI. Toluene oxidation in a dielectric barrier discharge combined with heterogeneous catalysis. Romania J of Phys Supplement P 2011; 56:156-162.
- Sultana S, Vandenbroucke, AN, Leys C, De Geyter N, Morent R. Abatement of VOCs with Alternate Adsorption and Plasma-Assisted Regeneration: A Review. Catalysts 2015; 5:718-746; doi:10.3390/catal5020718
- Wu J, Xia Q, Wang H, Li Z. Catalytic performance of plasma catalysis system with nickel oxide catalysts on different supports for toluene removal: Effect of water vapor. Appl. Cat. B 156–157 (2014) 265–272.
- Vandenbroucke AM, Morent R, Geyter ND, Leys C. Non- thermal plasmas for non-catalytic and catalytic VOC abatement. J of Hazard mater 2011; 195:30-54.
- Subrahmanyam C, Renken L, Kiwi-Minsker L. Novel catalytic non-thermal plasma reactor for the abatement of VOCs. Chem Eng J 2007; 134:78-83.
- Sekiguchi H. Catalysis assisted plasma decomposition of benzene using dielectric barrier discharge. Canadian J of Chem Eng 2001; 79:4 512-516.
- Ogata A, Shintani N, Mizuno K, Kushiyama S, Yamamoto T. Decomposition of benzene using a non-thermal plasma reactor packed with ferroelectric pellets. IEEE Trans on Ind Appl 1999; 35:753-759.
- Zhang X, Chen W, Zhu J, Feng W, Yan K. Aerosol Formation and Decomposition of Benzene Derivatives by AC/DC Streamer Corona Discharge. Int J of Plasma Environ Sci and Technol 2010; 4:2 130-134.
- van Hest MFAM, de Graaf A, van de Sanden MCM, Schram DC. Use of in situ FTIR spectroscopy and mass spectrometry in an expanding hydrocarbon plasma. Plasma Sources Sci and Technol 2000; 9:615-624.
- Chen LH, Lee MH, Chen HS, Chuang BM. Review of packed bed plasma reactor for ozone generation and air pollution control. Ind and Eng Chem Res 2008; 47:2122-2130.
- Abdullahi ME, Abu Hassan MA, Zainura ZN, Raja Ibrahim RK, Integrated air stripping and non thermal plasma system for the treatment of Volatile organic compounds from wastewater: statistical optimization.. Desal. and water Treat. 2015; doi: 10.1080/19443994.2015.1076353
- Ogata A, Ito D, Mizuno K, Kushiyama S, Yamamoto T. Effect of coexisting components on aromatic decomposition in a packed-bed plasma reactor. Appl Cat A: Gen 2002; 236:9-15.
- Takaki K, Urashima K Chang JS. Scale-up of ferro-electric packed bed reactor for C2F6 decomposition. Thin solid films 2006; 506-507:414-417.
- Takaki K, Takahashi S, Mukaigawa S, Fujiwara T, Sugawara K, Sugawara T. Influence of pellet shape of ferroelectric packed bed plasma reactor on ozone generation and NO removal. Int J of Plasma Environ Sci and Technol 2009; 3:1 28-34.
- Zhu T, Wan Y, Li H, Chen S, Fang Y. VOCs decomposition via modified ferroelectric packed bed dielectric barrier discharge plasma. IEEE Trans on Plasma Sci 2011; 39:8 1695-1700.
- Kumar S. Spectroscopy of Organic Compounds. National Science Digital Library at NISCAIR, India 2006; Retrieved on 21st April, 2014 from http://nsdl.niscair.res.in/bitstream/123456789/793/1/spectroscopy+of+organic+compounds.pdf
- Kohno H, Tamura M, Shibuya A, Honda S, Berezin AA, Chang JS Yamamoto T. Destruction of volatile organic compounds used in a semiconductor industry by a capillary tube discharge reactor. IEEE Trans on Ind Appl 1998; 34:5 953-966.
- Liang W, Li J, Li J, Jin Y. Abatement of toluene from gas streams via ferroelectric packed bed dielectric barrier discharge plasma. J of Hazard Mater 2009; 170:633-638.
- Chang C, Lin T. Decomposition of toluene and acetone in packed dielectric barrier discharge reactors. Plasma Chem Plasma Process 2005; 25:3 227-243.
- Ikaunieks J, Mezmale L, Zandeckis A, Pubule J, Blumberga A, Veidenbergs I. Non-thermal Plasma for VOC Treatment in Flue Gases. Scientific J of Riga Tech University: Environ and Climate Technol 2011; 6:31-37.
- Kuroki T, Hirai K, Kawabata R, Okubo M, Yamamoto T. Decomposition of Adsorbed Xylene on Adsorbents Using Non thermal Plasma with Gas Circulation. IEEE Trans on Ind Appl 2010; 46:2 672-679.
- Hakoda T, Matsumoto K, Mizuno A, Hirota K. Catalytic effect of Pt and Ag-loaded γ-Al2O3 on the oxidation of xylene in air at low temperature under electron-beam irradiation. Int J of Plasma and Environ Sci and Technol 2010; 40:1 65-70.
- Holzer F, Kopinke FD, Roland U. Influence of ferroelectric materials and catalysts on the performance of non-thermal plasma (NTP) for the removal of air pollutants. Plasma Chem Plasma Process 2005; 25:6 595-611.
- Yu-fang G, Dai-qi Y, Ke-fu C. Toluene removal characteristics by a superimposed wire plate dielectric barrier discharge plasma reactor. J of Environ Sci 2006; 18:2 276-280.
- Li J, Zhu T, Fan X, He W. Decomposition of dilute VOCs in air by streamer discharge. Int J of Plasma and Environ Sce and Technol 2007; 1:2 141-144.
- Kim H, Ogata A, Futamura S. Decomposition of Gas-Phase Benzene Using Hybrid Systems of a Non-Thermal Plasma and Catalysts. J of the Korean Physical Soc 2004; 44:5 1163-1167.